Introduction
Non-alcoholic fatty liver disease (NAFLD) is an escalating and challenging global health problem. It has a complex pathogenesis pathway and lacks of satisfactory drug therapy. NAFLD may progress to non-alcoholic steatohepatitis (NASH), liver cirrhosis, and hepatocellular carcinoma. Nutritional factors, insulin resistance, oxidative stress, inflammation, and genetic factors are involved in the development of NAFLD [1-3].
A plethora of drugs such as insulin sensitizers, antioxidants, and lipid-lowering drugs have been tried as potential therapies for NAFLD, all without a satisfactory outcome [4]. Hence, optimizing the therapeutic strategy for NAFLD remains a medical challenge.
Relying on the multisystem effects of NAFLD, combination therapy should be considered in planning the treatment strategy. Among the suggested promising drugs for treatment of NAFLD are metformin and vitamin E. Metformin, an insulin sensitizer, has been shown to decrease fat deposition and inhibit the inflammatory responses in hepatocytes [5]. The role of vitamin E in the treatment of NAFLD is based on its activity as an antioxidant [6].
A growing body of evidence has demonstrated the association between the serum level of uric acid and the presence of NAFLD [7]. Hence, the current study aimed to compare the effect of the triple combination between uric acid lowering agent, allopurinol, plus metformin and vitamin E versus their respective monotherapy or double therapy on prevention of NAFLD in rats.
Material and methods
Animals
The current study was conducted on male albino rats weighing 200-250 g. Rats were housed in metallic cages and allowed free access to tap water and standard rat chow diet for 2 weeks before the start of the experiment. The facilities were maintained at 24°C ±2°C with a 12-h dark: light cycle. Animals were treated according to the ARRIVE guidelines and in accordance with the U.K. Animals Act, 1986 and accompanying guidelines, and EU Directive 2010/63/EU for animal studies. The experiment was adherent to the ethical guidelines of the local ethical committee and approved by the board of the Faculty of Medicine, Minia University, Egypt.
Drugs and chemicals
Metformin and allopurinol were obtained as powders from Cid CO., Egypt. Vitamin E oil was obtained from El Hekma CO., Egypt. Metformin and allopurinol powders were dissolved in distilled water and freshly prepared before administration. Vitamin E oil was dispersed in distilled water.
Induction of fatty liver
Fatty liver was induced by adding 10% fructose to the drinking water for 4 consecutive weeks [8].
Experimental design
Rats were divided into 7 groups of 6 animals each: normal control that received vehicle; positive control model group that was given 10% fructose in drinking water and received no treatment; allopurinol group that was treated with allopurinol 50 mg/kg/day; metformin group that was treated with metformin 300 mg/kg/day; vitamin E group that was treated with vitamin E 100 mg/kg/day; metformin plus vitamin E group that was cotreated with metformin 300 mg/kg/day plus vitamin E 100 mg/kg/day; and allopurinol plus metformin plus vitamin E group that was co-treated with allopurinol 30 mg/kg/day plus metformin 150 mg/kg/day plus vitamin E 100 mg/kg/day.
The tested drugs were given orally by a stomach tube for 4 weeks from the first day of the study.
Sample collection and storage
At the end of the experiment, rats were weighed then sacrificed under anesthesia by using urethane (125 mg/kg i.p.). Blood samples were withdrawn from the abdominal aorta and centrifuged (5000 rpm for 10 minutes) for sera collection. The collected sera were used for the measurement of biochemical parameters. The liver was rapidly excised, cleaned of blood by saline, and weighed. For each rat, one part from the right lobe of the liver was kept in 10% formalin for histopathological evaluation, parts were cut in 5 µm thicknesses then deparaffinized and rehydrated for immunostaining and another part was accurately weighed and processed for biochemical measurements.
Biochemical measurements
Measurement of triglycerides in the serum and hepatic tissue
Triglycerides (TG) level in the serum was measured using an enzymatic colorimetric kit (catalogue NO: TG 119050) according to the manufacturer’s instructions. The indicator was quinoneimine that formed from hydrogen peroxide, 4-aminoantipyrine and 4-chlorophenol under the catalytic influence of peroxidases [9].
Extraction of lipids from the liver tissue was done using the Folch method. Equal parts from the liver were homogenized in chloroform/methanol (2 : 1) (1 γ in 20 ml). Then, the homogenate was centrifuged to recover the liquid phase. The solvent then washed by 0.2 volumes (4 ml for 20 ml) of 0.9% NaCl solution. After centrifugation for 10 seconds, the mixture was centrifuged again (2000 revolutions per minute) for separation of the two phases. The upper phase was removed and the lower chloroform phase containing lipids was used for measurement of TG using an enzymatic colorimetric kit (catalog number: 31400) according to the manufacturer’s instructions [10].
Determination of uric acid in serum
The level of uric acid in the serum was evaluated using the enzymatic colorimetric kit (catalog NO: UA119090). In this method, uricase enzyme oxidized uric acid to allantoin and hydrogen peroxide. The formed hydrogen peroxide led to oxidative coupling of 3,5-dichloro-2-hydroxybenzene sulfonate (DHBS) and 4-aminoantipyrine, in the presence of peroxidase, forming a red colored quinoneimine dye. The intensity of the color was directly proportional to the concentration of uric acid in the sample, with maximum absorbance at 550 nm.
Oxidative stress parameters in hepatic tissue
Hepatic tissue samples were homogenized in phosphate buffer solution, then the homogenate was centrifuged for 15 min and the supernatant was used for measurement of oxidative stress parameters: malondialdehyde (MDA), superoxide dismutase (SOD) and reduced glutathione (GSH).
Hepatic MDA content, a lipid peroxidation indicator, was measured chemically by the spectrophotometric method. This was based on the reaction between MDA and thiobarbituric acid as previously described elsewhere [11].
SOD activity was assessed chemically as previously measured by Marklund and Markuland (1974) [12]. The process was based on the fact that SOD inhibits the autoxidation of pyrogallol. One unit of SOD is generally defined as the amount of enzyme that inhibits the autoxidation of pyrogallol by 50%. The activity of SOD was measured at 420 nm.
Hepatic content of GSH was measured as previously described [13]. The protein in liver homogenate was precipitated with trichloroacetic acid and then centrifuged at 1000 rpm for 5 min. The reaction mixture containing 0.25 ml of supernatant, 1 ml of 0.2 M Tris–HCl (containing 1 mM EDTA, pH 8.9) and 0.05 ml of 10 mM 5,5’-dithiobis-(2-nitrobenzoic acid) in absolute methanol was kept at room temperature for 5 min, and the yellow color developed was monitored by a spectrophotometer at 412 nm.
Tumor necrosis factor α in hepatic tissue
Tumor necrosis factor α (TNF-α) was determined using an ELISA (Enzyme-Linked Immunosorbent Assay) kit (Sigma-Aldrich, St. Louis, MO, USA) (catalog NO RAB0480). Monoclonal anti-rat antibodies for TNF-α were precoated onto 96-well plates. Samples and standard were added to the wells followed by addition of biotinylated antibody to each well. Avidin-Biotin-Peroxidase Complex (ABC) was added and a substrate solution was used and produced a blue color that changed to yellow color after adding acidic stop solution. The density of the color was monitored at 450 nm immediately.
Histopathological examination of hepatic tissue
Liver tissue samples were fixed in formalin and embedded in paraffin. Four micron-thickness sections were taken, stained with hematoxylin and eosin stain and examined under a light microscope in a blind manner. The severity of liver steatosis was graded as 1, 2, 3, and 4 according to the extent of fatty cells as follows: 1 when fatty cells ≤ 25%; 2 when fatty cells = 26-50%; 3 when fatty cells = 51-75%; 4 when fatty cells > 75% of cells. Counting the number of inflammatory foci was used for scoring of the inflammation [14].
Immunostaining of iNOS in hepatic tissue
For evaluating the iNOS immunoexpression, 5 µm thicknesses from the right lobe of the liver were cut, deparaffinized in xylene and rehydrated in graded alcohol. Endogenous peroxidase activity was blocked using H2O/methanol, followed by adding citrate buffer (pH 6.0). Then, the sections were incubated at room temperature for 40 min with the anti-iNOS monoclonal anti-rat antibodies (Sigma-Aldrich, St. Louis, MO, USA).
Detection of the staining was done using UltraVision (Thermo Scientific) according to the manufacturer’s instructions. Scoring for immunostaining of iNOS was done by counting the number of stained cells using 5 high power fields for each rat [15].
Statistical analysis
Results were expressed as means ± standard error of mean (S.E.M.). One-way analysis of variance (ANOVA) followed by the Tukey-Kramer test was used to analyze the results for statistically significant difference. P values were considered to be significant if less than 0.05. GraphPad Prism was used for statistical calculations (version 5.01 for Windows, GraphPad Software, San Diego California USA, www.graphpad.com).
Results
Body weight and liver index
There was a significant elevation in liver index (liver weight/body weight) in the fructose model group as compared to the control group. Rats treated with allopurinol, metformin, vitamin E, metformin plus vitamin E and allopurinol plus vitamin E plus allopurinol showed a significant decline in the liver index compared to the model group (Table 1).
Table 1
Effect of allopurinol, metformin, vitamin E and their combination on liver index, and serum levels of liver enzymes, triglycerides, and uric acid
Group | Liver index Liver weight (mg)/body weight (g) | Serum TG (mg/dl) | ALT (U/l) | AST (U/l) | Uric acid (mg/dl) |
---|---|---|---|---|---|
Control | 2.28 ±0.19 | 91.50 ±5.98 | 25.02 ±2.87 | 21.20 ±3.78 | 3.00 ±0.36 |
Fructose (F) | 4.86 ±0.34* | 166.70 ±8.82* | 68.28 ±3.26* | 60.60 ±3.21* | 7.41 ±0.40* |
F/Allopurinol (A) | 3.74 ±0.11# | 110.32 ±7.13# | 37.67 ±2.14# | 40.60 ±2.50*# | 3.33 ±0.35# |
F/Metformin (M) | 3.56 ±0.29# | 109.70 ±3.73# | 43.10 ±3.94*# | 41.40 ±3.20*# | 5.50 ±0.45*# |
F/Vitamin E (E) | 3.94 ±0.19# | 94.00 ±7.07# | 43.92 ±3.97*# | 39.41 ±5.90*# | 4.72 ±0.25*# |
F/M + E | 3.02 ±1.02# | 94.67 ±6.76# | 34.67 ±1.23# | 30.60 ±1.66# | 5.25 ±0.30*# |
F/A + M + E | 3.66 ±0.21# | 79.17 ±7.08# | 25.47 ±3.63#$ | 22.61 ±1.25#$ | 3.50 ±0.42#$ |
Serum activities of alanine aminotransferase and aspartate aminotransferase
Serum activities of ALT and AST were significantly elevated in the fructose control group as compared with the normal control group. Groups treated with allopurinol, metformin, vitamin E or metformin plus vitamin E showed a significant amelioration in serum ALT and AST activities compared to the fructose group. Rats treated with a combination of metformin plus vitamin E plus allopurinol showed a further significant improvement in ALT and AST activities compared to other treated groups (Table 1).
Serum level of uric acid
In the fructose control group, serum uric acid was significantly elevation compared to the control group. Groups treated with allopurinol, metformin and/or vitamin E showed a significant decline in serum uric acid compared to fructose group and other groups. Rats treated with the triple combination (allopurinol/vitamin E/metformin) revealed a further significant decline in serum level of uric acid compared to either treated group (Table 1).
Triglycerides in serum and hepatic tissue
Serum and hepatic levels of TG were significantly elevation in the fructose control group as compared with the normal control group. Treatment with allopurinol, metformin, vitamin E and their combination showed a significant amelioration in serum and hepatic TG level compared to the fructose group (Tables 1 and 2).
Table 2
Effects of allopurinol, metformin, vitamin E and their combination on oxidative stress parameters and triglycerides in hepatic tissue
Group | GSH (mg/g tissue) | SOD (U/g tissue) | MDA (nmol/g tissue) | TG (mg/g tissue) |
---|---|---|---|---|
Control | 129.7 ±1.14 | 1539 ±32.62 | 81.24 ±5.61 | 89.77 ±7.85 |
Fructose (F) | 107 ±1.70* | 1224 ±69.01* | 128.73 ±2.53* | 213.70 ±10.33* |
F/Allopurinol (A) | 119.3 ±2.77*# | 1481 ±19.18# | 101 ±1.46*# | 90.80 ±3.31# |
F/Metformin (M) | 128.9 ±1.44# | 1548 ±38.68# | 98.23 ±2.83*# | 105.90 ±4.34# |
F/Vitamin E (E) | 122.9 ±1.76# | 1502 ±42.13# | 101.44 ±3.87*# | 92.20 ±3.48# |
F/M + E | 122.5 ±1.67# | 1502 ±31.66# | 92.83 ±2.24# | 101.8 ±3.07# |
F/A + M + E | 129.8 ±1.30# | 1530 ±41.14# | 91.26 ±1.76# | 97.09 ±4.04# |
Oxidative stress parameters in hepatic tissue
There was a significant reduction in hepatic tissue content of GSH and the activity of SOD in the fructose group compared to the control group. Treatment with allopurinol, metformin and/or vitamin E, and their combination with allopurinol significantly increased hepatic content of GSH and activity of SOD levels compared to the fructose group. Hepatic content of MDA significantly increased in the fructose group and significantly decreased it in rats treated with metformin and vitamin E and their combination with allopurinol (Table 2).
Tumor necrosis factor α in hepatic tissue
In the fructose control group, there was a significant increase in hepatic tissue content of TNF-α compared with the control group. Treatment with allopurinol, metformin and/or vitamin E and their combination with allopurinol significantly decreased hepatic TNF-α level as compared to the fructose group (Fig. 1).
Histopathology of hepatic tissue
Histopathological examination (Fig. 2 and Table 3) revealed hepatic architecture in the normal control group (A). In the fructose control group (B), there was a significant increase in liver steatosis and inflammation as compared to normal control rats. Treatment with allopurinol (C), metformin (D), vitamin E (E) or metformin plus vitamin E (F) caused significant attenuation of steatosis compared to the fructose group. Rat treated with the combination of metformin plus vitamin E plus allopurinol (G) showed a significant improvement in liver steatosis and inflammatory foci as compared to either the fructose group or other treated groups (Fig. 2, Table 3).
Table 3
Effect of allopurinol, metformin, vitamin E and their combination on histopathological score of hepatic tissue
Group | Steatosis | Inflammation |
---|---|---|
Control | 0.00 ±0.00 | 0.00 ±0.00 |
Fructose (F) | 3.0 ±0.00* | 1.66 ±0.33* |
F/Allopurinol (A) | 1.45 ±0.01*# | 1.45 ±0.01* |
F/Metformin (M) | 1.66 ±0.32*# | 1.00 ±0.00* |
F/Vitamin E (E) | 2.33 ±0.31*# | 0.66 ±0.33* |
F/M + E | 1.67 ±0.12*# | 1.012 ±0.04* |
F/A + M + E | 0.66 ±0.30#$ | 0.33 ±0.33# |
Fig. 2
Photomicrograph of the liver tissue: A) control group with normal liver histology, B) fructose group showing ballooning of hepatocytes with vacuolated cytoplasm and darkly stained nuclei (curved arrow), microvesicular steatosis (straight arrow), and inflammatory cell infiltration (star). C-E) Allopurinol, metformin and vitamin E treated groups, respectively showing mild (D,E) to moderate (F) improvement in liver histology, F) metformin plus vitamin E treated group showing moderate improvement in liver histology and G) combination group (allopurinol plus metformin plus vitamin E) showing significant improvement liver histology (most of the hepatocytes are back to normal (arrowhead). Note absence of inflammatory cell infiltration (H&E, scale bar 20 μm)
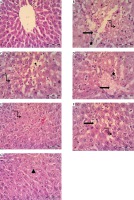
Immunostaining of iNOS in hepatic tissue
Figure 3 shows a significant increase in iNOS immunostaining in the model group (panel B) compared to the normal control (panel A). Rats treated with allopurinol (panel C), metformin (panel D), vitamin E (panel E) and metformin plus vitamin E (panel F) revealed a significant decrease in iNOS immunostaining compared with the non-treated model group (panel B).The rats treated with the combination of allopurinol plus metformin plus vitamin E (panel G) showed a significant decrease in the immunostaining of iNOS compared to other treated groups.
Fig. 3
iNOS immunostaining: A) negative expression of iNOS in liver tissue of (B) positive iNOS immunostaining in numerous cells (arrow) of liver tissue of fructose treated group. Note that the expression is mainly in nonparenchymal cells. C-E) panels show a decrease in the number of immunopositive cells in allopurinol, metformin and vitamin E treated groups, respectively. Note: low intensity of immunostaining. F) shows very few positive cells in metformin plus vitamin E treated group. G) Panel shows nearly negative expression of (INOS) in liver tissue of combination group (allopurinol plus metformin plus vitamin E); hematoxylin counterstain, scale bar 20 μm. H represents the score of iNOS expression (number of positive stained cells) in hepatic tissue. Data are presented as mean ± SEM. #,*,$significant compared to normal control, fructose non-treated, and either monotherapy or double therapy treated groups, respectively
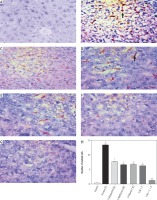
Discussion
Hyperuricemia plays a crucial role in the development of NAFLD [7, 16]. The present study presents a new finding that triple therapy with allopurinol plus metformin plus vitamin E showed higher efficacy in prevention of fructose-induced fatty liver than either the individual therapy or the double therapy with vitamin E plus metformin.
In this study, fructose-induced NAFLD was manifested by an elevation in the serum activities of liver enzymes (ALT and AST), indicating injury in liver cells. The damaged liver cells caused leakage of these cytoplasmic enzymes into the circulation [17, 18]. Development of NAFLD was confirmed by histopathological examination in the form of microvesicular steatosis and inflammation accompanied with an increased liver index [17, 19].
The study elucidated the effect of the tested drugs on the pathogenesis of fructose-induced fatty liver by measuring metabolic and oxidative stress and inflammatory parameters.
In this study, fructose fed rats (model group) revealed an increase in serum and hepatic levels of TG along with a high serum level of uric acid [20, 21].
Several explanations have been suggested for the association between TG and fructose-induced fatty liver. First, fructose is a lipogenic factor that leads to generation of TG as a direct product of its metabolism [22]. Second, chronic consumption of high fructose stimulates the secretion of apolipoprotein B48 with subsequent stimulation of intestinal synthesis of free cholesterol, and TG. Third, fructose, unlike glucose, does not enhance the release of insulin and leptin, which are considered the main factors that regulate energy production and adiposity [22].
Hyperuricemia is crucial in the development of fructose-induced fatty liver, and there is increasing evidence indicating that an elevated uric acid level represents a trigger for NAFLD development [23]. In the liver, ketohexokinase enzyme metabolizes fructose in a process that utilizes ATP to phosphorylate fructose to fructose-1-phosphate. Ketohexokinase enzyme, in contrast to hexokinases, which metabolize glucose, cannot cause a negative feedback effect. Consequently, there is no prevention of excessive phosphorylation with subsequent depletion of phosphate concentration inside the cell. Such an effect leads to stimulation of adenosine monophosphate deaminase, which converts adenosine monophosphate (AMP) to inosine monophosphate and eventually produces uric acid [23]. Hyperuricemia stimulates lipogenesis through induction of insulin resistance via direct inhibition of insulin receptor substrates. Additionally, hyperuricemia induces the inflammation and oxidative stress in liver cells [2, 24].
The present manuscript focused on the effect of allopurinol as an additional drug to the currently partially effective drugs (metformin and vitamin E).
Interestingly, the current results showed that rats treated with a combination of allopurinol plus metformin plus vitamin E caused a significant attenuation of NAFLD compared with either individual drug or double therapy (metformin plus vitamin E). This observation was evident in the histopathological examination as well as in the activities of liver enzymes. Such an effect was associated with significant attenuation of the serum level of uric acid. The hepatoprotective effects of allopurinol, vitamin E and metformin in NAFLD were reported in previous studies [25, 26].
As regard the signaling mechanisms, it has been reported that the stimulant impact of fructose on carbohydrate-responsive element-binding protein (ChREBP) was inhibited by using allopurinol [27]. The mechanism by which allopurinol blocks ChREBP nuclear translocation could be mediated by way of inactivation of AMP kinase, a recognized inhibitor of ChREBP activity [28]. Additionally, it has been reported that hyperuricemia induces insulin resistance (a principle pathway of NAFLD) via inhibition of phospho-insulin receptor substrate 1 (IRS1; Ser307), phospho-AKT (Ser473) signals and insulin-stimulated glucose uptake. Inhibition of uric acid synthesis reverses these signals, thereby preventing insulin resistance [29].
In NAFLD, oxidative stress is considered a key factor in hepatocellular injury. The mitochondrial respiratory chain is the main subcellular supply of reactive oxygen species, which may harm the mitochondrial proteins, lipids and mitochondrial DNA [30, 31]. In the current study, rats treated with allopurinol, metformin and/or vitamin E, and metformin plus vitamin E plus allopurinol showed a decrease in the level of MDA and improved GSH and SOD enzyme activities. A previous study was carried out on a rat model of diabetes where metformin was noted to ameliorate mitochondrial oxidative stress as manifested by a reduction in mitochondrial aconitase activity [32]. Vitamin E is a lipid-soluble antioxidant that is incorporated into cell membranes, protecting them from oxidative damage. It acts as a chain-breaking antioxidant and repairs oxidizing radicals directly by preventing the chain propagation step [33, 34].
The data of the present study, consistently with previous studies, showed a significant increase in TNF-α in NAFLD [35, 36]. This might be attributed to the ability of adipose tissue, an endocrine tissue, to produce some of the proteins termed adipokines, which include leptin, TNFα, interleukin (IL)-6, resistin, and adiponectin. Such substances play an important role in the inflammatory process and insulin resistance associated with NAFLD [36].
In the current study, treatment of rats with allopurinol, metformin and/or vitamin E attenuated fructose-induced liver injury as indicated by the improvement in the activity of liver enzymes (ALT and AST) and confirmed by histopathological examination of liver tissue as well as by a reduction in the liver index. Additionally, the tested drugs reduced serum levels of uric acid and hepatic tissue content of TG, along with improvement of oxidative stress parameters. These findings were in consistence with previous studies [37-40]. It has been reported that hyperuricemia and oxidative stress might release the inflammatory mediators including TNF-α and iNOS that participate in the pathogenesis of NAFLD-associated liver injury [39, 40].
The results of this study indicated that the tested drugs either alone or in combination mitigated the expression of iNOS in hepatic tissue. It is worth noting that the triple therapy with allopurinol plus vitamin E plus metformin revealed significant mitigation of iNOS expression as compared to either the individual or the double therapy regimen. The released iNOS leads to synthesis of a large quantity of NO, eventually with an increase in the oxidative product peroxynitrite. Inhibition of uric acid synthesis can inhibit overexpression of iNOS, leading to amelioration of excessive production of the end product NO. These effects were observed in the present study and in previous studies [16].
Conclusions
In summary, metformin, and vitamin E (either individually or in combination) showed a kind of protection against fructose-induced NAFLD. Their effects were associated with a reduction in oxidative stress parameters, TG, and the expression of TNF-α and iNOS in hepatic tissue. However, addition of allopurinol to vitamin E and metformin (i.e. triple therapy) caused a synergistic effect in prevention of fructose-induced NAFLD that was evident biochemically by liver enzymes (ALT and AST) and ascertained by histopathological examination. Such a synergistic effect was accompanied with a further significant reduction in the serum level of uric acid and hepatic iNOS immunostaining compared with other treatment regimens. The current results imply that the combination therapy between allopurinol plus metformin plus vitamin E is better in prevention of NAFLD than either individual or double therapy. It is worth noting that further large scale animal and human studies are needed to confirm the present findings.