Introduction
Cancer-related mortality represents the second leading cause of premature death globally, with its incidence rising steadily – from 5.6 million cases in 1990 to 10.8 million in 2019 [1]. In the United States alone, the year 2023 is expected to see 609,820 deaths and nearly 2.0 million new cancer diagnoses [2]. This escalating issue has catalysed a surge in the exploration of novel treatment strategies. A notable approach is the concept of drug repurposing, wherein established pharmaceuticals, previously utilised in distinct medical domains, are being reassessed for their efficacy, in this case in oncology. This methodology is particularly advantageous due to the well-documented safety profiles and the established spectrum of side effects of these drugs, a critical consideration given the typically high toxicity associated with oncological therapies [3]. In this realm, the antibiotic nitroxoline (NIT), initially introduced in the 1960s, has emerged as a compound of significant interest, demonstrating potential therapeutic benefits in the treatment of cancer [4]. Currently, the potential for NIT as a treatment option is being explored across various cancers, including ones originating from the bladder, prostate, pancreas, lungs, brain, and other sites [5–10]. This review investigates the use of NIT in urologic cancer treatment, discusses potential adverse effects, and digs into specific mechanisms of action within the framework of urologic oncology.
Material and methods
In this systematic review, we present a comprehensive analysis of the therapeutic potential of NIT in urologic oncology, underscored by strict adherence to the preferred reporting items for systematic reviews and meta-analyses (PRISMA) guidelines and the population, intervention, comparison, and outcome (PICO) framework. We formulated the following PICO question to guide our inquiry: In humans with cancer, animal models, and tumour cell lines (P), how does nitroxoline treatment, either alone or supplemented to existing regimens (I), compare to regimens without nitroxoline (C), in terms of the response to therapy in humans or any observed effects on tumour in animal models and cell lines (O)?
Two independent researchers conducted a systematic literature search of the PubMed, Medline, and Embase databases using the following search string: Nitroxoline AND (oncology OR cancer OR tumor OR tumour). The inclusion criteria were limited to original studies published in English language. Studies published in languages other than English, as well as reviews, conference reports, case-reports, letters to the publisher, and comments, were excluded. Additionally, a cross-reference search was carried out. The process of literature search and selection is transparently detailed in the PRISMA flow chart below (Fig. 1). The publication has been recorded in the international prospective register of systematic reviews (PROSPERO) and assigned the code CRD42024502266. To find as many reports of NIT adverse events (AEs) as possible, we performed additional searches for clinical trials unrelated to oncology.
Fig. 1
The preferred reporting items for systematic reviews and meta-analyses flow-chart presenting the entire literature search process
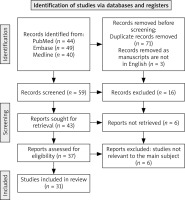
Safety of administration
Chemotherapy-associated adverse effects (CAAEs) resulting in a decline of quality of life are the main cause of discontinuation of effective regimens. Additionally, some CAAEs develop years after the completion of treatment. Others lead to infertility, neuropathies, secondary malignancies, and severe organ toxicities [11]. In light of the aforementioned, the development of novel treatment strategies must be balanced between efficacy and patient safety. In this context, a repurposing strategy is particularly enticing because most considered medicines have already been in use for decades. This is also the case with NIT.
The vast majority of clinical studies on NIT have been conducted in the context of urinary tract infections (UTIs). Naber et al. identified 32 clinical trials related to UTIs (4 of which were randomised and 28 were not) [12]. Of these, 29 reported on AEs. To this date, no severe AEs have been reported in various clinical settings [13].
Upon reviewing the outcomes of 4 high-quality, multicentre, single-blind randomised controlled trials that included 466 patients, Naber et al. found that 9.4% of participants undergoing NIT therapy encountered AEs, as opposed to 7.8% in the control group, although this difference was not statistically significant. Gastrointestinal AEs such as nausea, stomach pain, and diarrhoea were the most frequently reported. Headache, itching, and eyelid swelling were reported; however, these were isolated incidents. A total of 5 individuals terminated their treatment due to these AEs, with 3 from the NIT group and 2 from the control group [12].
Within the scope of 28 controlled and uncontrolled clinical trials, a total of 1354 individuals were involved. Among these, 6 trials involving 478 adult participants and 7 trials including 182 paediatric subjects revealed no complications. However, some AEs were reported in the remaining ones. Gastrointestinal complaints, particularly nausea, vomiting, and diarrhoea were the most frequently mentioned, with prevalence rates spanning 1.3–9% across trials. One study including 60 adults reported itching in 3.3% of cases. Exanthema was noted in 2.2% of participants in another study. In CTs conducted on children, nausea was the sole AE reported, affecting up to 10% of the paediatric population [12].
A solitary clinical case of NIT overdose has been identified. It holds significance, especially in the context of oncologic treatment regimens where in general higher dosages are administered. The aforementioned case involved an individual with suicidal intentions who consumed 5000 mg of NIT. Upon admission to the hospital, the patient exhibited only symptoms of fatigue while remaining alert and oriented. No further toxicity signs were noted. The patient recovered without the need for any specific medical intervention [14].
Bladder
Bladder cancer (BC) ranks as the 10th most common malignancy globally [15, 16] with data suggesting that 50–70% of on-muscle invasive bladder cancer (NMIBC) patients experience recurrence, and 30% progress to MIBC [10, 17, 18]. The 2023 European Association of Urology guidelines recommend transurethral resection of the bladder tumour followed by intravesical instillation of bacille Calmette-Guerin (BCG) therapy as the standard treatment for NMIBC. However, the effectiveness of BCG therapy is limited in some patients due to drug resistance or intolerable side effects [19]. Furthermore, some BCs already invade the muscle at diagnosis, complicating treatment strategies. Likewise, the resistance to standard chemotherapeutics like doxorubicin (DOX) and cisplatin [10]. The genomic heterogeneity of urothelial cancers is one explanation for these diverse responses and treatment failures, emphasising the need for novel drugs and their tailored utilisation. This also highlights the challenges in preventing high recurrence and addressing the progression to MIBC, which contribute significantly to incidence and mortality.
Angiogenesis
Many development and progression of human diseases rely on angiogenesis [20–23]. This is also the case with cancer. Its significance in tumour growth and metastasis is well understood; therefore, inhibiting angiogenesis has emerged as a promising technique for slowing tumour growth and increasing the efficacy of cytotoxic anticancer treatments [24, 25]. Shim et al. investigated the type 2 methionine aminopeptidase (MetAP2) pathway and sirtuins as the 2 most probable mechanisms for angiogenesis inhibition. Human umbilical vein endothelial cells (HUVEC), human BC KU7 line, and human breast cancer HCC1954 were evaluated.
The type 2 methionine aminopeptidase protein has been widely identified and extensively studied as a molecular target for angiogenesis inhibitors [26–29]. The type 2 methionine aminopeptidase inhibition activates p53, a tumour suppressor, and increases cyclin-dependent kinase inhibitor 1A. As a result, the cyclin E1 and cyclin-dependent kinase 2 (CDK2) complex, which phosphorylates retinoblastoma 1 (RB1) and regulates endothelial cell G1-S cell cycle transition, are inhibited [30, 31]. Moreover, increasing evidence links silent information regulators (sirtuins) to cellular ageing and angiogenesis [32]. In vivo, SIRT1 suppression causes endothelial cell senescence and prevents blood vessel development [33, 34].
Shim et al. performed 2 concurrent screening assays: one targeting MetAP2 with a 175,000-compound chemical library, and another utilising HUVEC against the Johns Hopkins Drug Library [35]. These screens were aimed at discovering new inhibitors of angiogenesis. Substances indicated by both drug libraries were designated for further testing. One of the most potent double hits was NIT. Firstly, scientists cultured HUVEC with different concentrations of NIT to verify its potency of proliferation inhibition. Secondly, to verify the mechanism of action (MoA) of NIT, small inter-interfering RNAs (siRNAs) targeting MetAP2 and SIRT1 were transfected into HUVEC. Changes in p53, p21, and RB1 protein expression were assessed. The results of the study group matched the positive control. Nitroxoline demonstrated potent and highly selective inhibition of MetAP2. Additionally, a dose-dependent inhibition of SIRT1 and SIRT2 activity was observed when treated with NIT. Conversely, NIT exerted no changes in SIRT3.
Following the experiments on cell lines, an in vivo Matrigel plug assay was performed on mice. One group received a carrier solution, the other NIT, both administered intraperitoneally once a day. After 3 days of pre-treatment, a Matrigel plug containing pro-angiogenic factors was implanted subcutaneously near the abdominal midline. Treatment continued for an additional 7 days. Subsequently, the plugs were harvested and stained. Compared with the control, NIT significantly reduced the formation of new microvessels.
Next, Human breast cancer xenograft has been explored. HCC1954 cells were injected subcutaneously. Once the tumours were noticeable, the mice were grouped based on mass size to ensure equal average volume across the 2 groups. The first group received a carrier solution, and the other was treated with NIT, both administered intraperitoneally every other day. Mass volume was monitored throughout the study. After 30 days, the mice were euthanised and tumours were collected for analyses. Treatment with NIT resulted in a 60% reduction in volume and a 43% decrease in tumour weight compared to the controls. Furthermore, NIT therapy increased the acetylation of the TP53 protein in tumour tissues, indicating an inhibition of SIRT1 activity. Furthermore, the levels of MetAP2 substrate Met-14-3-3 as well as p53, p21, and RB1 were elevated, implying that NIT suppresses this pathway activity in vivo. Immunohistochemical analysis revealed a 58% reduction in angiogenesis in the NIT-treated group.
Lastly, an orthotopic BC model was employed. KU7 cells were orthotopically injected into murine bladders. Three days later, the mice were treated orally with either NIT or placebo for 2 weeks. Multiple bioluminescence imaging showed that NIT treatment significantly reduced tumour growth compared to the placebo. The histopathological analysis further demonstrated a noticeable decrease in tumour size in the NIT group. Immunoblot analysis of bladder tissues was consistent with in vitro results. No significant difference in SIRT1-related protein levels was detected between groups, and acetylated TP53 (acetyl-K382) levels were undetectable in both groups, precluding further analysis of TP53. These differences certainly highlight the cell line-specific MoA of NIT and emphasise the necessity for additional investigation.
Drug resistance
Drug resistance is a significant challenge in cancer treatment, with about 80–90% of cancer-related deaths being directly or indirectly linked to it [36]. Additionally, nearly all drugs used in oncology have encountered some form of resistance, undermining the effectiveness of chemotherapy regimens [36]. One of the most frequently described mechanisms of resistance is drug efflux from the site of action. It has been described in most types of cancer and it is believed that the ATP-binding cassette transporter protein family is responsible for it [37–40].
Lin et al. explored this mechanism of resistance through the prism of BC, identifying overexpression of P-glycoprotein (P-gp) as the root cause [10]. Encoded by the MDR1 gene, it reduces intracellular concentrations of its substrates, such as DOX, by actively transporting them out of tumour cells, leading to decreased drug efficacy [41].
Evidence suggests that inhibiting P-gp may also increase CIS sensitivity. This implicates P-gp involvement in reducing tumour cell sensitivity to non-P-gp substrates (like CIS) [42]. Lin et al. also suggested that STAT3 influences MDR1 gene expression [10]. Additionally, a growing body of research describes an association between the overexpression STAT3 and BC poor prognosis. Therefore, STAT3 inhibition has been suggested to effectively downregulate P-gp expression [43, 44].
In his study, Lin et al. conducted both in vitro and in vivo investigations demonstrating the reversal of P-gp overexpression mediated by NIT application [10]. Scientists utilised human BC cell line T24, alongside DOX-resistant (T24/DOX) and CIS-resistant (T24/CIS). The T24 and T24/DOX cells were exposed to increasing concentrations of DOX, whereas T24 and T24/CIS cells were treated with CIS to evaluate the impact of NIT on cancer proliferation. This involved treating all cell lines with growing concentrations of NIT. The analysis encompassed drug sensitivity, cell cycle distribution, apoptosis examination, and western blot analysis. In animal xenograft models, tumour growth and body weight were monitored, followed by the harvesting of tumours for histological analysis.
A dose- and time-dependent reduction in viability was observed in the T24 cells and the drug-resistant lines upon treatment with NIT. Notably, as the NITs concentration increased, a higher proportion of cells remained in the G0/G1 phase across the T24, T24/DOX, and T24/CIS cells. Additionally, the percentage of cells undergoing apoptosis increased with the NIT concentration, reaching up to 62% in the drug-resistant lines. Western blot analysis revealed a dose-dependent suppression of STAT3 signalling and P-gp expression.
In the following section of the study, a subcutaneous xenograft model was employed in mice utilising T24/DOX and T24/CIS cells. The experimental groups received an oral course of NIT therapy at a dosage that the authors declared to be derived from the standard dosage for UTIs [45]. The control received phosphate-buffered saline. Notably, NIT treatment resulted in significant tumour size reduction, with corresponding decreases in average tumour weights. Tumour growth rates were slower in the NIT groups, with no significant changes in the body weight of the mice, indicating low regimen toxicity. Histological analysis showed distinct morphological changes, including cancer cell necrosis in the NIT group. Western blotting confirmed reduced levels of phosphorylated STAT3 (the most active form), P-gp, and Mcl-1 in NIT-treated tumours, but the change in total STAT3 expression was not statistically significant.
Reversing epithelial mesenchymal transition
Metastatic BC remains a formidable challenge in clinical oncology, marking a transition to an advanced stage associated with severely reduced survival rates. Furthermore, treating metastatic BC presents a considerable challenge due to the heterogeneity of metastatic lesions. Among these, numerous “private” mutations exist, and they keep evolving individually. Therefore, understanding the underlying mechanisms of metastasis development is of utmost importance [46]. Medical fitness for palliative chemotherapy in metastatic BC is another major concern; repurposing drugs with low toxicity would raise treatability [47].
Mechanism verification
In 2020, Xu et al. carried out a study to investigate the MoA of NIT in BC [48]. They conducted in vitro cell line experiments (MBT-2 and J82) as well as an animal model with C3H/He mice. Although numerous NIT MoAs were identified, scientists attempted to verify one previously undescribed in BC, i.e. the one involving the blockade of epithelial-mesenchymal transition (EMT). The process is crucial in embryonic development, tissue fibrosis, wound healing, and notably in cancer metastasis [49, 50]. Some of the key molecules in EMT include matrix metalloproteinases (MMPs) such as MMP-2 and MMP-9, which promote cancer cell migration and extracellular matrix degradation.
During the initial stage of the study, reduced viability of BC lines was observed in both dose- and time-dependent manners. Furthermore, treatment with NIT led to noticeable apoptotic changes, such as chromatin condensation and nuclear fragmentation, as compared to controls. Next, transwell and wound-healing assays showed significant NIT efficacy in impairing cell migration.
In the following section of this study, the impact of NITs on the expression of proteins related to EMT was investigated. The analysis showed a decrease in the levels of N-cadherin, slug, and MMP-9 in both cell lines. Howe-ver, the reduction in MMP-2 expression was observed only in MBT-2 cells. Moreover, NIT treatment led to a decrease in the expression of Bcl-2, an anti-apoptotic protein, while increasing the levels of cleaved caspase-3 (C-caspase-3), a pro-apoptotic protein.
Lastly, mice with subcutaneously implanted BC cells were investigated. Two treatment arms received different NIT regimens via intragastric administration over 12 days. The higher NIT dose significantly reduced tumour volume and weight when compared with the controls. Tumour section immunohistochemistry showed that the higher-dose treatment arm led to larger necrotic areas within the tumours and decreased cell proliferation, as indicated by lower expression of Ki-67, a marker for proliferation, and CD31, an endothelial marker indicating blood vessel density. Additionally, MMP-9 expression, associated with tumour invasion and metastasis, was significantly reduced in the high-dose group compared to the controls.
Molecular pathway identification
In 2022 Ren et al. attempted to establish the mechanism accountable for the aforementioned EMT inhibition [19]. As confirmed by many non-coding RNAs, circRNAs in particular contribute to processes such as cell proliferation, apoptosis, and cancer metastasis. Their impact was evaluated on the following cell lines: T24, UM-UC-3, TCCSUP, and J82.
Consistent with the prior findings, all cell lines employed in the study exhibited decreased migration capability as well as impaired healing abilities after NIT treatment. Next, using a circRNA microarray, molecules that were co-expressed and upregulated across all treated cell lines were identified. Then, using specific primers, circNDRG1 was identified as the molecule consistently elevated across all 4 cell lines, thereby prompting its selection for further exploration.
Building on this, they created 2 unique siRNAs that specifically target the circNDRG1. This small inter-interfering RNAs mediated reduction of circNDRG1 was achieved in 2 types of BC cells: T24 and UM-UC-3. Such altered cells have been evaluated using transwell and wound-healing assays. Reducing circNDRG1 increased cell spreading abilities. To further validate this, a plasmid increasing the expression of circNDRG1 was introduced into the T24 and UM-UC-3 cells. The cells’ metastatic ability decreased, indicating the protective function of circNDRG1.
Lastly, the efficacy of NIT in mitigating cancer metastasis was evaluated using a murine model. After introducing UM-UC-3 cells, marked with a luminescent molecule, into mice via tail vein injection, the subjects were treated with NIT. The IVIS imaging system showed increased luminescence intensity in mice with reduced circNDRG1 levels, indicating a greater cancer spread than in the control group.
To completely understand the pathway behind circNDRG1 expression. Ren et al. examined whether it may sponge BC-associated miRNA. Starbase, CircInteractome, and CircBank prediction tools were used to anticipate which miRNA circNDRG1 would target. miR-520h was the only microRNA predicted by all 3 tools. Further, the transwell and wound-healing assay showed that miR-520h increased the migration of BC cells, suggesting that circNDRG1’s MoA is associated with miR-520h. The next step was to identify miR-520h’s target gene. Using predictions from four databases (miRmap, starBase, TargetScan, and miRDB), a list of 172 potential genes was established. Smad7, known to be involved in EMT, was selected. Subsequent investigations demonstrated that treating cells with NIT increased smad7 levels and inhibited EMT. Ultimately, reducing smad7 expression in BC cells counteracted the anti-metastatic effects of the miR-520h inhibition, demonstrating that miR-520h facilitates cancer spread by targeting smad7 and modulating the EMT pathway.
Prostate
Prostate cancer (PCa) holds the position of the second most diagnosed cancer worldwide [51]. Notwithstanding, from 2007 to 2014, there was a sharp decline in PCa incidence, largely due to changes in screening guidelines that led to reduced testing for prostate-specific antigen (PSA) and subsequent overdiagnosis reduction. However, since 2014, there has been an approximate annual increase of 3% in the overall incidence rates, with advanced-stage PCa incidence rates climbing by 5% each year [52]. Despite overdiagnosis and overtreatment being a major concern, metastatic PCa still presents as largely incurable and resistant to the latest regimens. Around 6% of new PCa cases are metastatic at diagnosis and frequently androgen independent [53, 54]. The magnitude of this issue is underlined by the fact that there has been no significant progress in increasing the survival rates for men with advanced PCa in the past 20 years [55]; as a result, NIT is now part of the repurposing inventory for PCa treatment.
Cell cycle arrest and apoptosis induction
The 2015 study by Chang et al. delves into the potential of NIT repurposing as a novel treatment against both hormone-sensitive and hormone-refractory PCa cells targeting LNCaP, PC-3, and DU-145 cell lines [9]. The research focuses on how NIT impacts the AMP-activated protein kinase (AMPK)/mTOR signalling pathway and interacts with Chk2 activation in the fight against PCa.
AMP-activated protein kinase acts as a crucial cellular regulator, overseeing essential functions such as glucose absorption, fatty acid oxidation, and more [56, 57]. Its role in halting cancer cell growth by changing energy balance makes it a target for cancer therapy. Alongside Chk2, serine/threonine protein, a kinase activated in response to DNA damage, plays a fundamental role in the cellular DNA damage response [58, 59], either facilitating DNA repair or enforcing cell cycle arrest and triggering apoptosis, preventing the spread of genetically compromised cells [60, 61].
The researchers discovered that NIT triggers G1 arrest of the cell cycle and subsequent death of PCa cells via an AMPK-dependent mechanism [9]. Nitroxoline was demonstrated to block both the mTOR-p70S6K signalling pathway and the cyclin D1-Rb-Cdc25A axis. Furthermore, NIT triggered AMPK-dependent activation of Chk2, which was followed by a shift from pro-survival DNA repair activity to pro-apoptotic one. This at least partially contributes to cancer cell death. Overall, NIT inhibited cell growth in hormone-sensitive and hormone-refractory cell lines in a time-dependent manner. Furthermore, NIT-treated cell lines demonstrated increased autophagy rates, as shown by a rise in the well-known autophagy-related protein LC3-II [62]. This shows that NIT may play a role in the development of therapies for PCa.
To further exclude possible confounding effects on PCa cells exerted by NIT’s chelating abilities, additional investigations have been conducted. Iron and zinc – cofactors in hundreds of processes which regulate the cell cycle – might be influenced by NIT’s chelating ability. This is particularly useful in eliminating bacterial infections [63]. A theory assuming the influence of chelating properties on the viability of PCa cell lines was also verified. The anticancer action of NIT was found to be unaffected by ZnCl2 supplementation. As a result, NITs MoA in PCa cells were shown to be independent of its ability to chelate ions.
PD-1 blockade and nitroxoline synergy
In the 2019 research conducted by Xu et al., the efficacy of combining NIT with PD-1 blockade therapy in the treatment of PCa was explored [64]. This investigation involved in vitro studies using human and murine PCa cell lines, as well as in vivo studies within orthotopic animal models. The cell lines used were human PCa lines LNCaP, DU145, and PC3, and the normal epithelial prostate cell line RWPE-1. Additionally, the study incorporated the murine PCa cell line RM9. The RM9 cells were genetically modified to express luciferase and human PSA, creating the RM9-Luc-PSA cells. This particular cell line is advantageous for dynamically tracking drug responses and tumour progression.
PD-1 receptor, known as programmed death 1, is a receptor located on the surface of T-cells, playing a crucial role in the immune system’s checkpoint mechanisms [65]. It functions to prevent autoimmune reactions by controlling the intensity of immune responses, thus minimising potential tissue damage [66]. Programmed death-ligand 1 (PD-L1) is a ligand that binds to the PD-1 receptor. It is found on the surface of various cell types, including some cancer cells. The interaction between PD-1 and PD-L1 leads to the suppression of T-cell activity, allowing tumours with high PD-L1 expression to evade the immune system’s attack [67]. This evasion facilitates the growth and spread of the tumour by shielding it from immune-mediated destruction. Concluding, PD-L1 expression is associated with a poor prognosis, adverse outcomes, and higher recurrence rates [68–71].
In PCa murine models, Xu et al. examined the effectiveness of treatments using NIT, PD-1 blockade, and a combination of both (NIT + PD-1 blockade). Through in vivo imaging, it was found that the effectiveness of NIT matched that of PD-1 blockade when compared to a control group. Additionally, reductions in serum PSA levels were observed in each treatment arm, with the most significant decreases seen in the NIT + PD-1 blockade. This treatment also resulted in the lowest tumour size and weight. Furthermore, examination of histological sections from resected tumours revealed that microvascular density was notably reduced in the group receiving the dual-drug treatment. The findings suggest that combining NIT and PD-1 blockade enhances the anti-tumour response across all evaluated treatment effects.
In the in vitro analyses, NIT notably decreased the viability of PCa cell lines RM9-Luc-PSA, LNCaP, DU145, and PC3 over time. On the other hand, no significant cytotoxic effects were observed in normal RWPE-1 cells. Subsequent investigations focused on NIT’s impact on cell cycle progression and apoptosis. Nitroxoline treatment altered the expression of key cell cycle regulatory molecules, leading to cell cycle arrest in the analysed PCa lines. Specifically, NIT reduced the levels of CDK2, CDK6, and cyclin D3 in a dose-dependent manner. The absence of these molecules results in the arrest of the cell cycle [64]. Moreover, researchers discovered that NIT also lowers the levels of Bcl-2 and Bcl-xL while enhancing the expression of caspase-3 – proteins intimately linked to apoptosis. This pattern of expression supports the induction of apoptosis.
Conclusions
Drug repurposing is reshaping how new treatments are developed, significantly cutting the costs and time required to bring a new one to the market, while also leveraging the known safety profile of this medication. Quinolone derivatives, including NIT, have gained considerable interest in the field of oncology. The application of NIT in urologic oncology shows great potential. However, different cell lines have been shown to respond differently to NIT treatment. We believe that the next step in evaluating the effectiveness of novel, tailored therapies using NIT is to perform genomic profiling of numerous cell lines. This will involve identifying molecular profiles that can predict the success or failure of NITs in therapeutic application. Similar research initiatives are currently examining new pharmaceuticals and certain types of cancer [72–74]. In parallel with the development of other innovative drugs, this approach could substantially enhance patient treatment outcomes through targeted therapy regimens based on detailed molecular profiling of their disease.
To provide a comprehensive overview of the role of NIT in urological oncology, it is important to acknowledge that certain studies focused on NIT derivatives were not covered by this review. Notwithstanding, these substances yielded encouraging results when used to study renal cell carcinoma [75]. Furthermore, NIT is currently being evaluated in a multicentre phase II clinical trial for NMIBC, registered under the trial identifier CTR20131716. Taking it all into consideration, we believe it is just a matter of time before NIT or one of its novel derivatives gains traction in oncology.