Introduction
Asthma is estimated to affect more than 300 million individuals worldwide. Despite the efficacy of inhaled corticosteroids and long-acting β2 agonists, 5-10% of the patients have a persistent severe uncontrolled disease [1, 2]. Studies aimed at providing a mechanistic understanding of processes leading to asthmatic exacerbations are important as uncontrolled disease results in an increase in the health care burden and significant mortality [1, 3]. Viral respiratory infections, particularly with rhinovirus, are the most common triggers of asthmatic exacerbation [4]. Other causes are allergen sensitization and pollutants such as tobacco smoke, ozone, and particulate matter [4, 5].
Asthma has been categorized in four or five endotypes that are determined by factors such as age of onset, allergy prevalence and genetic predisposition [6, 7]. If presenting beyond the age of 20 asthma is of late onset [8]. Among the endotypes, late onset asthma is associated with two: T2 and non-T2. T2 patients are not as allergic as patients with early-onset asthma, but the endotype is signified by blood eosinophilia [7]. The release of toxic proteins such as eosinophil cationic protein (ECP) from eosinophils can confer damage to the respiratory epithelium and a high level of eosinophil granulocytes has been linked to exacerbations [9-11]. Therapy targeting the eosinophil promoting cytokine IL-5, such as mepolizumab, reduced the eosinophil cell count and also exacerbations in patients with the T2 endotype and eosinophilia [12]. T helper type 2 (Th2) and innate lymphoid cells type 2 (ILC2) produce IL-4, IL-5 and IL-13 in the T2 endotype [13, 14]. The anti-IL-4 antibody dupilumab has shown benefit for patients with T2 asthma [15]. Fraction of exhaled nitric oxide (FENO) is also used to identify T2 disease [7]. IgE bound to mast cells and crosslinked by allergen induces degranulation of proteins such as tryptase [16, 17]. Patients with T2 asthma are more prone to exacerbations in response to rhinoviral infection than non-T2 patients [4, 14]. Genome-wide association studies of asthmatic patients have identified linkage with the gene for the IL-33 receptor IL1RL1/ST2 [6]. The antibody itepekimab, targeting the alarmin IL-33, showed benefit for patients with severe asthma [18]. In experimental asthma, anti-IL-33 altered the composition of airway cells, decreasing myofibroblast numbers and mucus-producing cells and favouring ciliated epithelial cells [19]. The genetic association does not indicate T2 or non-T2 preference. Pneumovirus-induced release of the alarmin IL-33 from bronchial epithelium can activate both T cells and innate type 2 cells during asthmatic exacerbations [14]. In T2 asthma there is a weakened ability to fight pneumovirus, which might be because the innate immune response is defective; IgE-bound plasmacytoid exhibited an impaired interferon (IFN)-α response [4]. IL-33 also reduced IFN-β levels, preventing a Th1 promoting dendritic cell phenotype while the Th2 response was unaffected [20].
Late onset non-T2 asthma, on the other hand, occurs independently of allergen exposure [7]. Triggers for this type of the disease overlap with T2 and include endotoxins, viral and bacterial infection, cigarette smoke and other occupational agents. Studies have shown that non-T2 patients have bacterial flora of higher diversity and with a higher burden than T2 patients [20, 21]. Non-T2 disease can be driven by CXCL8 (IL-8) production and neutrophil infiltration, leading to bronchial hyperresponsiveness and steroid resistance [10]. Furthermore, Th17 cells have been associated with the non-T2 endotype. Released IL-17 can promote neutrophil infiltration, muscle cell proliferation and exacerbations [22, 23]. Efforts to reduce neutrophils and IL-17 in patients with severe non-T2 asthma by targeting the CXCL8 receptor CXCR2 (AZD5069) and the IL-17 receptor A (brodalumab) were however unsuccessful [24, 25].
The induction of chronic inflammation and the modulation of innate and adaptive immune processes in environmentally exposed tissues are regulated by IL-31, which plays a crucial role. In addition, IL-31 triggers the production of proinflammatory cytokines and matrix metalloproteinases [26]. According to recent evidence, IL-31 may be implicated in the promotion of allergic inflammation and an airway epithelial response associated with allergic asthma. There was a significant correlation between the IL-31 single nucleotide polymorphisms (SNPs) and total serum levels of IgE in patients diagnosed with asthma [27]. Moreover, research indicated that IL-31 had a substantial impact on the elevation of epidermal growth factor (EGF) levels within a transformed human bronchial epithelial cell line [28].
Several studies have furthermore indicated that the categorization of adult-onset asthma into specific T2 and non-T2 endotypes might be an oversimplification. Itepekimab and dupilumab did not together synergistically improve asthma, suggesting that complete inhibition of one immune pathway might induce another since immune responses cross-regulate each other [18]. In an animal model of asthma, inhibition of the Th2 response similarly induced a Th17 response but inhibition of both IL-13 and IL-17 was required to reduce eosinophilia and asthma in this situation [29]. Our previous work showed that eosinophils can also be present in lungs of asthmatics with non-T2 disease [10]. A more detailed analysis of BALF immune cells from asthmatics using scRNAseq did not find specific T2 and non-T2 cell types but instead clustered patients into those with predominance of innate cells expressing the IL-4 receptor and those with a mixed population of Th1, Th2 and Th17 cells, CD4+ and CD8+, some being resident memory cells [30]. The aim of this study was to investigate the distinctive endotype marker profile among severe asthma patients hospitalized for exacerbations, with a specific emphasis on discerning the impact of viral versus non-viral triggers.
Material and methods
Patients
A cross-sectional study conducted at a general teaching hospital in Sulaimani city, Iraq recruited 49 asthma patients from July to December, 2022. The inclusion criteria were a diagnosis of asthma based on Global Initiative for Asthma (GINA) 2021 [31] and hospital admission due to disease exacerbation. Exclusion criteria were other causes of dyspnoea such as heart failure, renal failure of other pulmonary diseases such as chronic obstructive lung disease, pulmonary hypertension, and parenchymal lung disease. Patients had prior to admission for exacerbation been diagnosed as having mild persistent, moderate persistent, intermittent or severe asthma in accordance with GINA 2021 [31].
In our study, the diagnosis of asthma exacerbation was confirmed based on a combination of clinical symptoms, physical examination findings, and relevant laboratory tests. Clinical symptoms such as wheezing, coughing, dyspnoea, and chest tightness were primary indicators. These symptoms align with the classic presentation of asthma exacerbation as outlined in various clinical guidelines, including those from the GINA 2021 [31] and the National Asthma Education and Prevention Program (NAEPP) Expert Panel Report [32].
Furthermore, physical examination findings consistent with acute exacerbation, such as prolonged expiratory phase, diminished breath sounds, and the presence of accessory muscle use, were also considered diagnostic clues. Laboratory tests including spirometry, peak expiratory flow (PEF) measurements, and arterial blood gas analysis were employed to assess the severity of exacerbation and confirm the diagnosis.
The classification of asthma severity in our study is based on the Global Initiative for Asthma (GINA) 2021 guidelines. According to these guidelines, asthma severity is categorized into four levels: mild persistent, moderate persistent, intermittent, and severe.
Mild persistent: This category indicates asthma symptoms that occur more than twice a week but less than once a day, and night-time symptoms occur more than twice a month.
Moderate persistent: Asthma symptoms in this category occur daily, and night-time symptoms occur more than once a week.
Intermittent: Asthma symptoms occur less than twice a week, and night-time symptoms occur less than twice a month.
Severe: This category is characterized by asthma symptoms that are continuous throughout the day, limiting physical activity, and frequent night-time symptoms.
For comparison, 51 healthy controls were recruited to the study, none having symptoms of asthma or atopy. All the patients with allergic asthma tested positive (≥ 3 mm) for at least one common allergen on the skin prick test. Among the allergens were birch, timothy grass (Phleum pratense), mugwort (Artemisia vulgaris), cat, dog, horse, house dust mite (Dermatophagoides pteronyssinus), Cladosporium, and Alternaria. The skin prick test was negative for all the healthy controls (HC) and non-allergic asthma patients. Prior to being admitted to the hospital, only 33 out of 49 asthmatic patients (67.3%) used inhaled cortisone, as shown in Table 1. All participants – patients and healthy controls – consented to participate in the study. The study was approved by the Ethics Committee of the College of Medicine at the University of Sulaimani.
Table 1
Characteristics of patients with exacerbations and healthy controls
Blood collection and analysis
Five millilitres of blood was drawn from all participants. A small sample of the blood was used to detect absolute eosinophil count and for detection of ECP positive cells using immunohistochemistry. The Cytometry Kit for Detecting Eosinophil in Human Blood was used according to the manufacturer’s instructions (Orphee, France; catalogue number: 130-092-010). The remaining blood samples were transferred to appropriate tubes, stored at room temperature for 30 min and subsequently centrifuged to retrieve serum. Samples were stored at –70°C until further analysis. Optical flow measurement of immunoglobulin IgE in serum was performed using an ECLIA kit according to the manufacturer’s instructions (Roche, USA; catalogue number: 04827031190). IL-17 (catalogue number: E-CL-H0104, sensitivity range: 12.50-800 pg/ml), IL-31 (catalogue number: E-EL-H5469, sensitivity range: 7.81-500 pg/ml), IL-33 (catalogue number: E-EL-H2402, sensitivity range: 15.63-1000 pg/ml), ECP (catalogue number: E-EL-H1379, sensitivity range: 39.06-2500 pg/ml) and tryptase (catalogue number: E-EL-MK0594, sensitivity range: 31.25-2000 pg/ml) was determined in the serum samples using ELISA kits according to the manufacturer’s instructions (Elabscience Biotechnology Inc. Houston, USA).
Immunocytochemistry of peripheral blood
Blood samples were cytocentrifuged transferring white blood cells to slides. The slides were rinsed with BSA containing PBS and then incubated with an anti-ECP antibody (clone EG2) as previously described [33] (Diagnostic Development, Sweden; catalogue number: EG2). The ECP-antibody complex was visualized by using an alkaline phosphatase–anti-alkaline phosphatase (APAAP) kit with fast red substrate (DAKO, USA, catalogue number: IR779). Any unspecific binding of the AAP-antibody was determined by the omission of the primary antibody. The stained slides were analysed using a Leica DRMB microscope. Fuji 200 film was used for image capture.
Viral detection via nasopharyngeal swab
The nasopharyngeal swab was collected and placed into the viral transport medium (VTM) for transportation to the laboratory. Upon nucleic acid extraction, viral diagnosis was determined by interpreting the cycle threshold (Ct) values obtained from reverse transcription polymerase chain reaction (RT-PCR) analysis. The RT-PCR assay targeted a panel of respiratory viruses, including adenovirus, influenza virus, coronavirus, metapneumovirus, parainfluenza virus, respiratory syncytial virus, and rhinovirus based on the Polymerase Chain Reaction Respiratory Viral Panel Testing kit (Louisiana Healthcare Connections, USA, reference number: LA.CP.MP.181; CPT codes: 87632).
Statistical analysis
The normality tests (Shapiro-Wilk, Kolmogorov- Smirnov, De-Agostino) in GraphPad 9 Prism (GraphPad Software, USA) were performed to determine whether the data were normally distributed. As the data passed normality tests, we applied parametric tests for all data. Data were presented as mean ± standard error of the mean (SEM). The t-test was used for comparison of the two groups. The Pearson correlation test was used to assess the correlation between the variables. The ROC curve tested parameters that differentiated severe and non-severe cases of asthma. A ROC curve in MedCalc 20 (MedCalc Software, Belgium) provided values for area under the curve (AUC), cut-off, specificity, and sensitivity. P-values are indicated at the following significance levels: p < 0.05 = *, p < 0.001 = **.
Results
Patient characteristics
The characteristics of the 49 patients with asthmatic exacerbations and the 51 healthy controls are shown in Table 1. The average age of the patients was 50.4 ±12.9 years (range 16-101), not significantly different from the healthy controls (50.0 ±19.4, range: 17-80). About 60% of patients and healthy controls were female. As the average age of disease diagnosis was 42.8 ±16.1, and there was only one patient aged below 20, the majority of the patients had adult-onset asthma. The average duration of disease was 7.7 ±10.8 years, 24.5% of the patients being atopic and 26.5% having family history of asthma. The cause of exacerbation was reported to be due to infection or to uncontrolled asthma. Only one of the patients and none of the healthy controls were smokers. Therapy in the form of inhaled cortisone was utilized by 67.8% of patients. According to the GINA 2005 classification, five had mild persistent asthma, 23 moderate persistent, two intermittent and 14 severe asthma.
Analysis of inflammatory markers
The patients’ blood eosinophil level was significantly higher than healthy controls’ (0.3 ±0.26 vs. 0.11 ±0.14 × 109 cells/l, p = 0.003, Fig. 1A). The asthmatics had furthermore a higher level of the eosinophil protein ECP in serum than the healthy controls (45.7 ±4.2 vs. 15.6 ±9.9 µg/l, p = 0.0001, Fig. 1B). Blood cells were assessed for presence of ECP using immunohistochemistry (Fig. 1C, D). In agreement with findings from determination of blood eosinophil numbers and ECP measurement in serum, many cells stained for ECP, being eosinophils, among the blood cells in asthmatic individuals (Fig. 1C, representative example). IgE and the mast cell marker tryptase were determined in serum from the asthmatic patients and healthy controls (Fig. 1E, F). Both markers were higher in the group of asthmatics compared to the healthy controls (Fig. 1E, IgE: 360 ±52.5 vs. 11 ±131 IU/ml, p = 0.0001, Fig. 1F, tryptase: 48 ±3 vs. 19.3 ±13.2 µg/ml, p = 0.0001).
Fig. 1
Number of eosinophils present in the blood, ECP, IgE and tryptase in the serum of patients with asthmatic exacerbations and in healthy controls. The study included 49 patients with asthma exacerbations and 51 healthy controls. A) The number of eosinophils (cells/109 blood cells) present in the blood, the level of (B) ECP (μg/ml) in serum; C) Representative example of asthmatic patient’s white blood cells stained for ECP using anti-ECP antibody EG2; D) Asthmatic patient’s white blood cells but with omission of primary antibody; levels of (E) IgE (IU/ml) and (F) tryptase (μg/ml) in serum. Samples were taken from patients at the time of hospital admission and from healthy controls when available. Calculations to determine differences between groups were undertaken using Student’s t test and the respective significance levels are shown in the figure
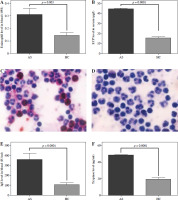
Interleukin 17 and IL-33 were higher in the patients’ serum compared to the control group (Fig. 2A, IL-17: 11.65 ±12.33 vs. 4.47 ±4.1 µg/ml, p = 0.0005, Fig. 2B, IL-33: 8.6 ±7.1 vs. 3.5 ±3.1 µg/ml, p = 0.0002). A higher level of IL-31 was also found in the asthmatic group than in the control group (Fig. 2C, 15.3 ±9.4 µg/ml) compared to healthy controls (7 ±5 µg/ml, p = 0.0001).
Fig. 2
Levels of IL-17, IL-33 and IL-31 detected in serum of patients with asthmatic exacerbations and in healthy controls. The study included 49 patients with asthma exacerbations and 51 healthy controls. The figure shows the level of A) IL-17, B) IL-33 and C) IL-31 in the serum at the time of hospital admission. Inflammatory mediators were also determined in the serum of healthy controls. Calculations to determine differences between groups were undertaken using Student’s t test and the respective significance levels are shown in the figure
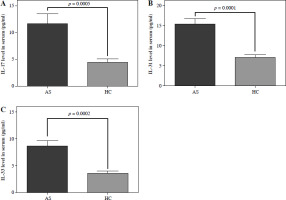
Correlations between markers of disease and disease severity
Correlation analyses were undertaken to compare the patients’ levels of inflammatory markers and disease severity based on symptom score. There was a statistically significant correlation between ECP and tryptase levels (r = 0.69, p = 0.0001). No significant correlation was however found when comparing ECP or tryptase with IgE level (Table 2). A correlation was found between IL-17 level and ECP (r = 0.35, p = 0.024), tryptase (r = 0.32, p = 0.039) and IgE levels (r = 0.50, p = 0.0006). IL-17 level was found also to correlate with IL-33 (r = 0.95, p = 0.0001) and IL-31 levels (r = 0.89, p = 0.0001) (Table 2). In terms of disease severity based on symptom score, there was a borderline significant correlation between tryptase level and symptom score (r = 0.30, p = 0.05).
Table 2
Correlations between inflammatory marker levels and symptom score
Assessing severity in asthmatic patients through biomarkers with ROC curve
By evaluating potential biomarkers, the severity of asthma can be determined using the ROC curve (Fig. 3, Table 3). Mild and severe cases of asthma can be distinguished by calculating the AUC of biomarkers. IgE is the most reliable biomarker for distinguishing severe and non-severe asthmatic patients, with the highest AUC value of 0.915. IL-17 and IL-31 follow with AUC values of 0.802 and 0.791, respectively. The recorded cut-offs were > 151.7 (IU/ml), > 68 (pg/ml), and > 45.6 (pg/ml), respectively. Nevertheless, the other biomarkers listed in Table 1 were not considered adequate for identifying asthma severity.
Fig. 3
Assessing severity in asthmatic patients through biomarkers (A – eosinophils, IgE, IL-17, and IL-31; B – IL-33, tryptase, and ECP) with ROC curve. The ROC curve analysis was plotted using MedCalc 20. Statistical significance was established with a p-value of less than 0.05
ECP – eosinophil cationic protein, IL – interleukin, IgE – immunoglobulin
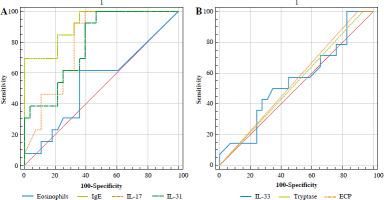
Table 3
Assessing severity in asthmatic patients through biomarkers with ROC curve
Viral detection among asthmatic patients
Among the eleven asthmatic patients studied, the following viruses were detected: respiratory syncytial virus in 2 cases (18.18%), rhinovirus in 3 cases (27.27%), influenza virus in two cases (18.18%), parainfluenza virus in one case (9.09%), adenovirus in one case (9.09%), and human metapneumovirus in two cases (18.18%).
Investigating the differences in IL-17 and IL-31 expression in exacerbated asthma patients with viral and non-viral infections
The results presented in Table 4 indicate significant differences in serum IL-17 and IL-31 levels between exacerbated asthma patients with viral and non-viral infections. For IL-17, the mean level in patients with viral infections (138.01 ±41.61) was significantly higher compared to those with non-viral infections (88.92 ±14.42) (p = 0.026), suggesting that viral infections may contribute to elevated IL-17 levels in exacerbated asthma. Similarly, for IL-31, the mean level in patients with viral infections (271.43 ±81.83) was markedly higher compared to those with non-viral infections (129.04 ±20.93) (p < 0.0001), indicating a substantial elevation in IL-31 levels during viral infections in exacerbated asthma cases.
Discussion
In this study, we characterized disease markers of patients with asthma, admitted due to exacerbations, to determine whether they can be classified into T2 or non-T2 endotype. The patients were found to have higher levels of T2 endotype markers, blood eosinophilia, ECP present in eosinophils, eosinophil degranulation of ECP, a high level of IgE, and the mast cell marker tryptase than those observed in the control group. In addition, IL-33, the Th2- associated IL-31 and the non-T2-associated IL-17 were elevated, suggesting that clear-cut T2 or non-T2 endotypes could not be discriminated in these patients.
Eosinophils and its granule protein ECP are markers of the T2 endotype. An elevated level of ECP has previously been shown to be associated with severe asthmatic disease [34]. Eosinophil dominated disease has been treated with anti-IL5, resulting in a reduction in exacerbations [12]. In a recent study of asthmatics, high ECP in the blood was found to be associated with chronic bronchitis and mucus production [35]. Although blood eosinophil levels are commonly used to discriminate the T2 endotype and direct treatment options, measurement of eosinophil granule protein release might best discriminate treatment response to mepolizumab [36]. Our previous research has however shown that eosinophils can also be present in the lungs of asthmatics with non-T2 disease [10]. ECP is stored in the mature secondary granules of eosinophils and can be released during asthmatic exacerbations. Although eosinophils are the major ECP producing cells, other cell types such as monocytes and neutrophils can produce or take up small amounts as well [37-39]. ECP is a cytotoxic ribonuclease, its physiological function being unknown, but its role in pathology being undisputed as it interacts with several lung-associated cell types [11].
We furthermore found that tryptase was the only inflammatory marker showing a correlation (borderline significant) with symptom score, potentially highlighting the involvement of mast cell degranulation during asthmatic exacerbations [16]. Tryptase-positive mast cells are elevated in human asthmatic bronchial tissue, where they localize to the epithelium, glandular, and bronchial smooth muscle regions [17]. The mast cell numbers correlate with airway hyper-reactivity, and tryptase levels in asthmatic bronchoalveolar lavage (BAL) fluid correlate with disease severity. A recent study reported that tryptase level in asthmatic subjects is independent of the T2 endotype, potentially providing an explanation to the findings in our study [16]. ECP measured in plasma correlated with tryptase, but there was no correlation with IgE. Tryptase also did not correlate with IgE, potentially reflecting the fact that only a third of the patients were atopic.
Further analysis of cytokine profiles indicated the involvement of a non-T2 innate response with production of IL-17 and correlation with other markers. Previous studies have identified the production of IL-17 and release of IL-33 during asthmatic exacerbations [14, 22]. Several studies have identified Th17 cell-driven asthma as a non-T2 endotype separate from T2 and associated with exacerbations [22, 23, 29]. Targeting of IL-17 has so far however not been successful in treating non-T2 asthmatic disease [25]. In our study, the level of IL-17 correlated with other inflammatory markers, including T2, such as levels of ECP, IgE and IL-33. Our findings thus suggest that adult-onset asthma cannot always be separated into clear-cut T2 and non-T2 endotypes.
The elevated levels of IL-17 and IL-31 in this research highlight the need to consider the role of infectious causes in asthma exacerbations. Viral infections seem to be linked to significantly higher levels of both IL-17 and IL-31, which could worsen the inflammatory response in these individuals. Viral infection with was the cause of at least some of the patients’ exacerbations in our study. A previous study involving adolescents showed that rhinovirus infection and serum IgE were associated with acute asthma exacerbations [40]. Another experimental study found that IL-33 and Th2 cytokines were induced during rhinovirus-induced asthma exacerbations. In this latter study virus-induced IL-33, as well as T cells and ILC2 cells responsive to the released alarmin, was the cause of the exacerbations [14].
The level of IL-31 was also increased during exacerbations and correlated with IL-17, ECP tryptase and IgE in our study. Studies have linked IL-31 to the T2 endotype [41]. IL-31 is produced by T cells, mast cells, eosinophils, dendritic cells and monocytes [42]. IL-4 upregulates IL-31 production in T cells [43]. The induction of bronchial hyperreactivity has been attributed to IL-31, a neuroimmune cytokine that signals through immune cells, epithelial cells, and the nervous system [44]. IL-31 and its receptor were found to be expressed in bronchial tissues of patients with severe asthma, but its expression was diminished in elderly patients [41, 45]. Further study of IL-31 in asthma endotypes is of interest.
Interestingly, recently inhibition of the epithelium derived thymic stromal lymphopoietin (TSLP) using tezepelumab improved asthmatic disease and increased FEV1 of both T2 and non-T2 patients, reducing the T2 markers eosinophils, FENO and IgE and IL-17. It was suggested that TSLP communication between immune cells and airway epithelial cells was inhibited, generally preventing inflammation [46]. Such therapy might be considered suitable for the patients in this study.
The ROC curve in this study showed differentiation of severe asthma individuals from non-severe asthma individuals using IgE, IL-17, and IL-31. Our results regarding IgE are consistent with the findings of Haselkorn and co-workers, 2010, who reported that IgE was linked to the severity of asthma [47]. Our findings on IL-17 as a potential severity biomarker align with those of Agache and Ciobanu, 2010, who observed that IL-17 could differentiate between severe and mild/moderate asthma cases. In their study, the cut-off for IL-17 was over 20 pg/ml, whereas in our current study, the cut-off is over 68 [48]. Our results differ from those of Ozceker and Bulut, 2018, who argued against the relevance of IL-31 in determining asthma severity [49].
Asthmatic patients subjected to biologic therapies have shown high variability of treatment response, which might be due to each individual’s underlying disease. Some patients show a very good response to treatment, while others do not. This suggests that endotype differentiation based on measurements of eosinophils, FeNO and other markers of disease do not provide sufficient information to provide the best treatment options. Although blood eosinophil levels are used to discriminate the T2 endotype, eosinophil activation status is not always known. Studies have suggested that measurement of eosinophil granule protein release might best discriminate treatment response, highlighting the potential importance of cell activation status [36]. That the asthmatic disease is highly individualised is highlighted by variability in factors such as epithelial oxidative stress, repair level, presence of embedded mast cell mediators and innate pathway activation. It has been proposed that the utility of omics strategies including immune cell and epithelial scRNAseq, metabolomics, proteomics and analysis using machine learning algorithms would result in a more personalized stratification [30, 50].
One of the limitations of this study is the absence of blood samples collected after the patients’ symptoms had improved. Our findings may not be applicable to asthmatic patients who are not experiencing exacerbations, which limits the generalizability of our results and hinders a comprehensive understanding of the larger asthma population. Additionally, a comparison between the viral and non-viral exacerbated asthma groups and the healthy control group is also missing. Another downside is the neglect of monitoring and continuing treatment for asthma exacerbations. The strengths of our study are that the patients were investigated during asthma exacerbations and that the panel of inflammatory markers covered both type 2 and innate inflammatory markers.
Conclusions
Patients with asthmatic exacerbations were found to have higher levels of both T2 and non-T2 inflamma- tory markers than healthy controls. The results further indicate a contribution of a Th17 cell and innate immune response potentially augmented by IL-33. The discovery of increased IL-31 levels during asthma flare-ups is a new finding that necessitates additional research. The study examined the differentiation of IgE, IL-17, and IL-31 levels in patients with severe and non-severe asthma. Additionally, the latter two cytokines could differentiate between exacerbated asthma resulting from viral infection and exacerbated asthma resulting from non-viral infection.