Introduction
Collagen is an abundant animal-derived protein that makes up approximately 30% of the total protein found in most organisms (Silvipriya et al., 2015). It is a crucial component of hair, skin, nails, tendons, and cartilage. Recently, there has been a growing interest among consumers in the dermatological (Barati et al., 2020) and orthopedic (García-Coronado et al., 2019) benefits of oral collagen supplementation. The popularity of collagen supplementation has increased not only for humans but also for animals, particularly dogs. Several recent studies have examined the positive effects of collagen supplementation in dogs, including during the treatment of osteoarthritis (Stabile et al., 2022) or joint diseases (Cabezas et al., 2022), as well as in healthy dogs during an exercise regimen (Varney et al., 2022).
The traditional sources of collagen are bovine and porcine skins and bones. However, the safety of collagen extracted from these animals is questionable due to the risk of disease transmission, such as bovine spongiform encephalopathy (Colchester and Colchester, 2005). To address this issue, researchers have turned to marinederived collagens, which can be extracted from fish byproducts, including skins, bones, and fins, in significant amounts and with increased biological safety (Yamamoto et al., 2014). While marine-derived collagens are a viable source for human supplementation and biomaterials, they are not always ideal for dogs to consume. As a result, there is still a need to search for new, nonmarine sources of collagen for use in dog supplementation.
Antlers, which are unique to cervids, consist of bone, cartilage, fibrous tissue, skin, nerves, and blood vessels. During the growth phase, antlers are unossified and cartilaginous, covered with highly vascular skin, called velvet, which supplies the growing bone with oxygen and nutrients. Once the antler has reached its full size, the cartilage is replaced with bone tissue and the velvet is lost (Price et al., 2005). Red deer (Cervus elaphus) antlers, which typically measure 71 cm and weigh 1 kg, are shed annually (Mattioli et al., 2022). In the pet industry, antlers are commonly used for dog chews because of two main reasons: 1) antlers are collected from the natural habitat without harming the animals and 2) antlers chews serve as a source of minerals, including calcium (Steiner-Bogdaszewska et al., 2022; Tajchman et al., 2022), and collagen (Rucklidge et al., 1997). We hypothesized that red deer antlers would be a good source of edible collagen for dogs. Therefore, our study aimed to verify the collagen content and molecular characterization of collagen in red deer antlers.
In recent decades, numerous studies have focused on optimizing the conditions for collagen extraction from various sources. However, very few have optimized the complete extraction procedure, including all three stages: alkaline, defatting, and acidic. Therefore, the objectives of this study were to fully optimize the collagen isolation protocol for antlers, to use this protocol for collagen extraction, and to characterize the collagen obtained from red deer antlers.
Materials and methods
Biological samples
Grounded antlers from red deer (Cervus elaphus) were obtained from TNC Pets company (Poznań, Poland, veterinary number PL 3021330p). All antlers were naturally shed by deer, collected from their natural open forest habitat, and utilized for the production of antler dog chews. The grounded antlers were stored in sealed plastic containers at room temperature. For collagen extraction, 800 mg of antler sample was used each time.
Experimental design
Multiple experimental designs were conducted to analyze the impact of chemical treatment concentration, temperature, and time on the extractability of collagen from red deer antlers. The extraction procedure involved three major steps: 1) removing noncollagenous proteins, 2) defatting, and 3) acidic extraction. This approach enabled the study of the influence of various factors on the efficiency of extracting acid-soluble collagen, including the temperature and time of the alkaline pretreatment (first step), the concentration and temperature of butyl alcohol during defatting (second step), and the concentration of acetic acid and time (third step). To cover a broad range of conditions and obtain values that maximize collagen isolation while reducing bioproduction times and concentrations, the values of independent variables were selected from previously reported studies. The total number of samples was 324 (108 for each of the three optimization steps) to maximize collagen extraction efficiency.
Alkaline pretreatment experimental design
In the pretreatment experimental design, independent variables were studied under specific conditions. These variables included a temperature of either 4 or 25°C and time intervals of 12, 24, or 36 h (Table 1). Previous studies in the literature had used time values ranging from 1 to 36 h, with a preferred temperature range of 4–25°C for the removal of noncollagen proteins. The antler:alkaline solution ratio was kept constant at 1 : 10 (w/v), and high agitation was maintained at 200 rpm. Following alkaline treatment, the grounded antler was washed with distilled water until the pH of the washing water reached a neutral level.
Defatting experimental design
To defat the samples, a 10% butyl alcohol solution was used at a solid-to-solvent ratio of 1 : 10, 1 : 15, or 1 : 30 (w/v) for 24 h at either 4 or 25°C. The antler samples were then washed with distilled water and lyophilized. The solid : solvent ratios during defatting commonly used in previous studies ranged from 1 : 10 to 1 : 30, with a preferred temperature range of 4–25°C.
Acid extraction experimental design
The collagen extraction stage of the experimental design involved studying independent variables under specific conditions. These variables included an antler-to-acetic acid ratio of 1 : 10, 1 : 15, or 1 : 30 (w/v) and time intervals ranging from 12 to 36 h. The temperature was kept constant at 4°C. Previous studies in the literature commonly used time values ranging from 1 to 32 h and a solid-to-acetic acid ratio of 1 : 10 to 1 : 20. Following collagen extraction, the solutions were collected, measured, dialyzed against 0.1 M acetic acid, and freeze-dried.
SDS-PAGE characterization of acid-soluble collagen
Samples with a concentration of 1 mg/ml were prepared in 1 × Laemmli sample buffer, denatured for 5 min at 100°C, and then applied to 6.5% polyacrylamide sodium dodecyl sulfate (SDS) gels. To determine the molecular weight of the samples, a molecular weight marker (Page-RulerTM Prestained Protein Ladder, 10–180 kDa, Thermo Fisher) was also loaded alongside the collagen samples. After electrophoresis, the gels were stained with silver following the method described by Kumar (2018).
Determination of denaturation temperature
The denaturation temperature (Td) of the collagen was determined using the method described by Kimura et al. (1988). A viscometer (Bohlin Visco) was filled with a 0.1% (w/v) collagen solution in 0.1 M acetic acid. The temperature was then increased incrementally from 20 to 35°C, and the samples were maintained at each temperature for 30 min. Fractional viscosities were calculated for each temperature, and the relative viscosity was determined with the viscosity obtained at 20°C. Thermal denaturation curves were created by plotting fractional viscosities against temperature. The Td was taken as the temperature at which the change in viscosity was half completed, corresponding to a fractional viscosity of 0.5.
Determination of UV-Vis spectra
Antler collagen was subjected to UV-Vis absorbance measurements using a Nicolet Evolution 220 PC UV-Vis spectrophotometer, following the method described by Abdollahi et al. (2017). A spectrum was recorded in the wavelength range of 190–220 nm.
Amino acid characterization of acid-soluble collagen
The amino acid composition of the freeze-dried collagen was determined using acid hydrolysis. The collagen was hydrolyzed with 6 N hydrochloric acid containing 0.1% phenol under an inert atmosphere and heated to 110°C for 24 h. The hydrolysate was then subjected to vacuum removal of HCl. An automated amino acid analyzer (AAA T-339, Mikrotechnika Praha Co) was used to analyze the hydrolysate. Amino acids were identified by derivatization with ninhydrin and measurement of absorbance at 570 nm, except for proline (Pro) and hydroxyproline (Hyp), for which absorbance at 440 nm was measured. The amino acid content was expressed as the number of a specific amino acid per 100 amino acid residues.
FTIR spectroscopy
Fourier transform infrared (FTIR) spectroscopy was used to determine the functional groups present in the extracted collagen. A Metler Toledo ReactIR 15 spectroscope was used in the range of 4000–650 cm-1. The sample was prepared by mixing 10 mg of lyophilized collagen with potassium bromide and forming a disc by pressing it into a translucent pellet using a hydraulic press. The mode of vibrations and functional groups were identified based on the peak of interest at a specific wavelength and absorbance, following the method described by Chen et al. (2016).
Results
Isolation of collagen from red deer antler
The yield of isolated collagen from red deer antler varied between 6.4 and 176.3 mg, depending on the treatment parameters at each step of the process (Table 2).
Table 2
The amount of collagen isolated from the red deer antler dust
Duration of alkaline pretreatment influences the collagen yield
During alkaline pretreatment, one of the two independent variables tested, i.e., temperature, had no significant effect on the amount of collagen recovered from the red deer antler dust (Table 2 and Fig. 1A). The median amount of isolated collagen using 25°C during alkaline pretreatment was 83.07 mg, which was comparable to the 84.00 mg obtained when the pretreatment was performed at 4°C. However, the duration of this step had a significant impact on collagen recovery, with 95.04 mg of collagen isolated when the alkaline pretreatment was conducted for 12 h, 88.81 mg for 24 h, and 64.47 mg for 36 h (Table 2 and Fig. 1B). Our findings suggest that a 12-h treatment was sufficient to condition the grounded antler for subsequent acid treatment, as it yielded the highest collagen extraction efficiency among the three-time durations tested. Furthermore, we observed that treatment durations of 24 or 36 h negatively affected collagen extraction from red deer antler. Therefore, the optimal variables for step 1 to maximize collagen recovery were 25°C and 12 h of treatment.
Fig. 1
Comparison of collagen yields isolated from red deer antler using different temperatures (A) and times (B) in step 1 (alkaline pretreatment); the violin plots show the frequency distribution of the data, with maximum and minimum values, median (central dashed line), and first and third quartiles (dense dashed bottom and top lines, respectively); significance was denoted as *P < 0.005, ***P 0.001
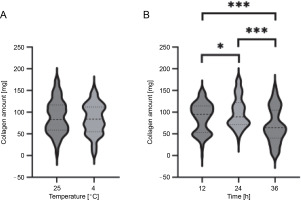
Longer defatting with higher antler to solvent ratio does not lead to higher collagen recovery efficiency
The next experiment focused on optimizing the defatting process by varying the time and antler-to-butyl alcohol ratio (Table 1). However, increasing both the processing time and the antler:solvent ratio did not significantly affect collagen recovery (84.52 and 82.36 mg of collagen were isolated at 25 and 4°C, respectively; 100.40, 104.46, and 109.60 mg were obtained at antler : solution ratios of 1 : 10, 1 : 15, and 1 : 30, respectively (Table 2 and Fig. 2). Therefore, due to industrial constraints such as the high cost of low-temperature processes and unnecessary usage of larger amounts of butyl alcohol, the optimal values of temperature and antler : butyl alcohol ratio were chosen as 25°C and 1 : 10, respectively. These consensus values were selected for collagen isolation from red deer antler.
Fig. 2
Comparison of collagen yields isolated from red deer antler using different temperatures (A) and grounded antler:solvent ratios (B) in the step 2 (defatting); the violin plots show the frequency distribution of the data, with maximum and minimum values, median (central dashed line), and first and third quartiles (dense dashed bottom and top lines, respectively)
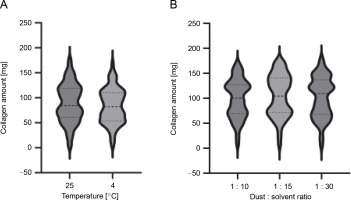
Longer acid extraction results in higher collagen yield
The acid extraction step involved two variables, i.e., time and antler to an acetic acid ratio (Table 1). Our results showed that increasing the time of acidic extraction from 12 to 36 h significantly increased collagen recovery (52.64 mg of collagen was extracted during 12 h compared to 123.15 mg during 36 h; Table 2 and Fig. 3A). However, the antler:solvent ratio did not have a significant effect on collagen recovery (111.60, 102.25, and 95.00 mg were obtained at antler : solution ratios of 1 : 10, 1 : 15, and 1 : 30, respectively; Table 2 and Fig. 3B). Therefore, the optimal values of duration and antler:acetic acid ratio were selected as 36 h and 1 : 10, respectively, for collagen isolation from red deer antler. These consensus values were determined based on the experimental results.
Fig. 3
Comparison of collagen yields isolated from red deer antler using different times (A) and grounded antler : solvent ratios (B) in the step 3 (acid extraction); the violin plots show the frequency distribution of the data, with maximum and minimum values, median (central dashed line), and first and third quartiles (dense dashed bottom and top lines, respectively); significance was denoted as ***P 0.001
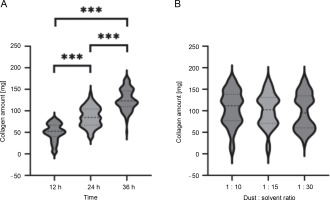
An optimized method of collagen isolation from red deer antler dust is highly repeatable
Based on the optimization of the three main steps of the collagen isolation procedure, we propose the following optimized conditions for the use with red deer antler: 1) removal of noncollagenous proteins at 25°C for 12 h, 2) defatting at 25°C using a grounded antler to butyl alcohol ratio of 1 : 10, and 3) acidic extraction for 36 h using a grounded antler to acetic acid ratio of 1 : 10.
To confirm the repeatability of the optimized method for collagen recovery from red deer antler, we performed collagen isolation in five technical replicates using the optimized conditions. The amount of isolated collagen from the red deer antler (171.40–176.30 mg) and the efficiency of collagen recovery (21.42–22.04%) are presented in Table 3. Our results demonstrate that the proposed method for collagen isolation from red deer antler dust is highly reproducible.
Table 3
The repeatability of the collagen isolation procedure from the red deer antler dust
Molecular characterization of red deer antler collagen reveals its typical features of type I collagen
Collagen profiling of the red deer antler was performed using SDS-PAGE (Fig. 4A). The electrophoretic patterns revealed the existence of the typical heterotrimer collagen structure containing two identical α1 chains (approximately 130 kDa) and one α2 chain (approximately 140 kDa).
Fig. 4
Molecular characterization of red deer antler collagen. (A) Sodium dodecyl sulfate-polyacrylamide gel electrophoresis (SDS-PAGE) patterns (S – collagen sample, M – molecular-weight marker); (B) Thermal denaturation temperature, (C) UV-Vis spectra, and (D) FTIR spectra
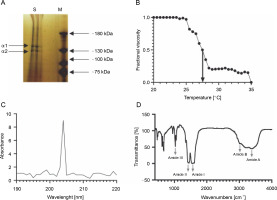
Table 4 presents the amino acid composition of red deer antler collagen. The major amino acid of collagen was glycine (31.7% of all amino acids), followed by alanine (6.7%). Contents of imino acids, such as Pro and Hyp were 33.90 and 18.7%, respectively.
Table 4
Amino acid composition of red deer antler-derived collagen
The thermal Td of the red deer antler collagen was calculated using the thermal denaturation curve. The Td was 27.5°C, as shown in Figure 4B.
Figure 4C presents the UV-Vis spectra of red deer antler collagen. The λmax was found to be 204 nm.
The FTIR spectra (400–4000 cm-1) of red deer antler collagen were analyzed and Figure 4D shows the major peaks (amide A, B, I, II, and III) with their assignments. The spectra exhibited significant absorption bands in the amide band region, including the peak of amide A at 3379 cm-1, amide B at 3010 cm-1, amide I at 1615 cm-1, amide II at 1555 cm-1, and amide III at 1400 cm-1.
Discussion
Previously published protocols for acid-soluble collagen extraction used a variety of sources, with fish waste material such as skin, scales, cartilage, and bones being the most common (Chen et al., 2016; Abdollahi et al., 2017; Ahmed et al., 2019; Blanco et al., 2019). However, only one study has investigated antler-derived collagen, which focused exclusively on velvet from elk (Cervus elaphus), rather than the mature antlers as a whole (Lee et al., 2014). As a result, most of the literature has relied on protocols established for fish material and did not include optimization studies. This study aims to contribute to the field by presenting an optimized protocol for collagen recovery from antlers. We have optimized all three major steps of the collagen isolation procedure, including prealkali treatment of antler, sample defatting, and collagen acidic extraction.
In this study, we focused on the extraction and characterization of collagen derived from red deer antler. Our results showed a collagen recovery rate ranging from 21.45 to 22.04%, which was significantly higher than the collagen recovery rate from elk antler velvet (5.2%) (Lee et al., 2014), fish waste materials such as skin, scales, and fins of Catla catla and Cirrhinus mrigala (5.8 and 4.7%, respectively) (Mahboob, 2015), Lutjanus sp. bones (4.5%) (Ramli et al., 2019), Atlantic cod (Gadus morhua) swim bladders (5.7%) (Sousa et al., 2020), scales of Cyprinidae (13.6%) (Chinh et al., 2019), and byproducts of bigeye tuna (Thunnus obesus) (13.5%) (Ahmed et al., 2019). The total yield of collagen was comparable to the amount of collagen obtained from the skin of several discarded fish species from the West Coast of the Iberian Peninsula (20%), including Chimaera monstrosa, Leucoraja naevus, Nezumia aequalis, Etmopterus spp., Galeus spp., and Scyliorhynus canicula (Sotelo et al., 2016). Furthermore, studies on Siberian sturgeon (Acipenser baerii) cartilage have also reported similar collagen amounts (27.13%) (Luo et al., 2018).
In this study, we observed that the electrophoretic positions of the α-chains for red deer antler-derived collagen were similar to those reported in previous studies (Lee et al., 2014; Mahboob, 2015; Sotelo et al., 2016; Ahmed et al., 2019; Chinh et al., 2019; Ramli et al., 2019; Sousa et al., 2020). However, in contrast to previous studies, we did not detect a clear band of the β-chain, and the band intensity of the α1 chain was not two-fold higher than that of the α2 chain. This finding, combined with the faint intensity of the β dimer, may suggest the presence of a higher crosslinking degree between the α2 chain in elasmobranchs, as previously proposed by Blanco et al. (2019).
The amino acid analysis of red deer antler collagen revealed that glycine was the major amino acid in the protein, accounting for approximately one-third of the total amino acids. This finding is consistent with previous studies that have shown glycine to be the major amino acid in type I collagen from different sources (Zhang et al., 2007; Sotelo et al., 2016; Luo et al., 2018; Ahmed et al., 2019). Alanine was also a prominent amino acid in red deer antler collagen (6.3%), and it was found to be the most abundant amino acid in carp scales and fins in two independent studies (Mahboob, 2015; Chinh et al., 2019).
Red deer antler collagen had high levels of Pro and Hyp, which are unique amino acids in collagen. Hydroxylation of Pro plays a crucial role in the thermal stability of the collagen helix through interchain hydrogen bonding via a bridging water molecule and direct hydrogen bonding with a carbonyl group (Wang et al., 2008). The contents of these two imino acids (as well as other amino acids) were very similar to those observed in elk antler velvet, as reported by Lee et al. (2014) (31.3 and 18.3%, respectively). In skin-derived collagens from different sources, the contents of imino acids were much lower, ranging from 6.6 to 8.3% Pro and 9.0 to 10.5% Hyp in C. catla (Mahboob, 2015), 8.3 to 12.1% Pro and 7.2 to 9.4% Hyp in several fish species from the Iberian Peninsula (Sotelo et al., 2016), and 15.0% Pro and 8.2% Hyp in tuna (Ahmed et al., 2019).
The levels of methionine and cysteine in red deer antler collagen were negligible, as was the case in C. catla and C. mrigala skin, scales, and fins (Mahboob, 2015). Rodziewicz-Motowidlo et al. (2008) also reported extremely low levels of methionine in C. mrigala skinderived collagen. Tryptophan and phenylalanine were not detected in the collagen isolated from red deer antler, but a small amount of tyrosine was identified, which may be attributed to the type of collagen (type I). Chen et al. (2004) and Mahboob (2015) have reported similar findings.
To determine the stability of the collagen, we conducted a thermal stability test, which measures the temperature at which the collagen transitions from its hydrogenbonded triple-helical structure to a random coil. The Td is primarily increased by crosslinks among the individual collagen molecules in the triple-helix (intrahelical crosslinking) and secondarily by intermolecular crosslinks.
Our study showed that the thermal Td of the extracted collagen from red deer antler was 27.5°C, which was significantly lower than the Td recorded for elk antler velvet (Td = 39.0°C) (Lee et al., 2014), but in a similar range to that of marine-derived collagens (29.6–30.0°C) (Ahmed et al., 2019; Chinh et al., 2019; Sousa et al., 2020). Previous studies have found that the thermal Td of collagen from animal species is correlated with the content of Pro and Hyp: the higher the imino acid content, the more stable the helical structure of the collagen molecule, and the Td is higher (De Simone et al., 2008). Therefore, we would have expected the Td of red deer antler collagen to be similar to that of elk antler velvet, but this was not the case.
The UV-Vis spectrum of red deer antler collagen showed an absorption peak at 204 nm, which is consistent with the findings of previous studies on UV-Vis spectrum analysis of type I collagen from elk antler velvet (200 nm) (Lee et al., 2014), carp fins (192.07 nm) (Chinh et al., 2019), Siberian sturgeon cartilage (230 nm) (Ahmed et al., 2019), and Atlantic cod swim bladders (221 nm) (Sousa et al., 2020). However, we did not observe clear peaks in the region corresponding to the aromatic amino acids, as suggested by the previously cited studies, and this was further confirmed by the amino acid composition analysis.
FTIR is a useful tool for evaluating and identifying the presence, chemical composition, and type of collagen. The FTIR reflectance spectrum of the extracted collagen indicated the presence of five major absorption peaks of amide groups: Amide A (N–H-stretching involved in hydrogen bonding), Amide B (asymmetrical stretching of CH2 groups), Amide I (C=O-stretching vibrations along the polypeptide backbone), Amide II (N–H bend coupled with C–N stretch), and Amide III (N–H bend). These results indicate that the analyzed collagen corresponds to type I collagen and that the functional groups present in the triple helix were not damaged by the extraction procedures. The absorption bands of red deer antler collagen were similar to those of other collagens (Zhang et al., 2007; Luo et al., 2018; Ahmed et al., 2019; Chinh et al., 2019).
Conclusion
In this study, we isolated acid-soluble collagen from red deer antlers using a holistically optimized method, and further characterized it using biochemical tools. Our findings suggest that red deer antlers could be a promising alternative source for collagen extraction, particularly as a substitute for collagen obtained from marine sources.