Introduction
The prevalence of hepatocellular cancer (HCC) is significant, as it is the fifth most prevalent and widespread cancer in the world (Chidambaranathan-Reghupaty et al., 2021). According to the International Agency for Research on Cancer report in 2020, liver cancer affected 905 700 people worldwide, and 830 200 people died from this disease. Hepatic cell damage caused by oxidative stress and inflammation is associated with hepatocarcinogenesis (Manosroi et al., 2015). Given the high worldwide prevalence of HCC, there is an urgent need to find new treatments to combat this multifactorial syndrome.
Surgical interventions (tumor resection and liver transplantation), percutaneous interventions (ethanol injection and radiofrequency thermal ablation), radiation therapy, drugs,and gene and immune therapies have significantly reduced liver diseases in HCC therapy. However, the lack of efficient in vitro models remains a significant challenge in discovering new therapies for hepatic carcinomas (Kumariet al., 2018). To reduce the morbidity and mortality of HCC, early diagnosis and the development of novel systemic therapies for advanced disease, including drugs, gene, and immune therapies, as well as primary HCC prevention, are crucial (Gosalia et al., 2017). Recent studies have focused on finding safe and effective natural anticancer medicines (Khan et al., 2020). Medicinal plants are the most promising source of novel chemical compounds with anticancer effects due to their successful clinical application.
Polyphenols, which comprise phenolic acids, flavonoids, and tannins, are a fascinating class of plant chemical compounds with anticancer characteristics (Cory et al., 2018; Lee and Lee, 2021). Antioxidants of plant origin have been suggested to possess favorable pharmacological effects such as antitumor, anticarcinogenic, and hepatoprotective effects due to their free radical scavenging properties, as suggested by several studies (Bahmani et al., 2016; Remila et al., 2015; Rozenblat et al., 2008).
Inula viscosa (Dittrichia viscosa) is a plant that belongs to the Asteraceae family and is native to the Mediterranean Sea. This plant has numerous beneficial effects on the body, including antimicrobial, antioxidant, antiulcerogenic, antihelmintic, and antidiabetic effects, as reported in several studies (Alkofahi et al., 1999; Chahmi et al., 2015; Hernández et al., 2007; Kheyar-Kraouche., et al., 2018; Oka et al., 2001; Talib et al., 2012). The leaves of I. viscosa have been used as a decoction in Algeria to treat various ailments, such as bronchitis and diabetes, as well as a cataplasm for injuries and rheumatic pain, according to studies (Baba Aissa, 2000; Haoui et al., 2015).
Phytochemicals were extracted from I. viscosa using solvents with varying polarities. In a previous study, Kheyar-Kraouche et al. (2018) identified 51 compounds in the ethanolic extract of I. viscosa leaves, including 11 phenolic acids, 23 flavonoids, one lignin, and 12 terpenoids. Of these compounds, 26 were described for the first time in I. viscosa. Additionally, several studies have reported on the anticancer effect of different extracts from various parts of this plant. Previous studies have indicated that extracts of I. viscosa and phytochemicals derived from this plant exert cytotoxicity against colon cancer (Bar-Shalom et al., 2019), cervical cancer (Virdi et al., 2020), breast cancer (Messaoudi et al., 2016), and melanoma cancer (Kamer Colak et al., 2021).
Building ontheprevious study, we aimed to investigate the impact of solvent polarity on the extraction efficiency of phenolic contents of I. viscosa leaves, as well as to evaluate the antioxidant activity, antiproliferative, and apoptotic effects on HepG2 liver cancer cells. Additionally, we assessed the affinity of the main phenolic compounds for binding to BCL-2, the antiapoptotic protein, using a molecular docking study.
Materials and methods
Plant material
The leaves of I. viscosa were collected from the Kharrata forest, which is situated at a distance of 58 km from Bejaia city in Algeria (coordinates: N 36°45′00″, E 5°04′00″″). The plant was identified botanically at the Laboratory of Plants Biotechnology and Ethnobotany, University Abderahmane Mira, Bejaia (Algeria). A voucher specimen was deposited there with the reference number IV017. The dried plant material was ground into a fine powder (with a diameter of 63 m) using an electric mill (Kika Labortechnik, Staufen, Germany), and then stored in a tightly closed container at 4°C for later use.
Extraction of phenolic compounds
To extract active compounds from the plant material, several solvents were utilized, including distilled water, ethanol (100%), ethanol (70%), ethyl acetate (100%), ethyl acetate (70%), chloroform (100%), and chloroform (70%). Each solvent was used to macerate 1 g of plant powder in 4 ml of the respective solvent at room temperature for 24 h. After centrifugation at 1500 g/min, the supernatant was retrieved, and the crude extract was obtained by drying. The extraction yield was calculated using the formula: yield% = weight of dried extract/ weight of the plant sample. Seven different extracts were collected and tested for various activities.
Total phenolic compounds, flavonoids, and condensed tannins determination
The total phenolic content was determined using the Folin-Ciocalteu technique (Kähkönen et al., 1999). Total flavonoid content was measured by the aluminum chloride colorimetric assay, as described by Maksimović et al. (2005). To determine the content of tannins, the vanillin technique adapted from Sun et al. (1998) was used.
Antioxidant activity
ABTS assay
The scavenging activity of the different extracts against the 2,2-azinobis (3-ethylbenzothiazoline-6-sulfonic acid (ABTS)) free radical was evaluated according to the method described by Re et al. (1999). ABTS+ was generated by combining 7 mM ABTS at pH 7.4 with 2.5 mM potassium persulfate (final concentration) and then storing it at room temperature for 16 h before use. The liquid was diluted with ethanol to obtain a solution with an absorbance of 0.70 ± 0.02 at 734 nm. For each sample, a diluted ethanol solution (100 μl) was allowed to react with a new ABTS solution (900 μl) for 6 min before measuring the absorbance. The results were reported as Trolox equivalent.
Ferric-reducing antioxidant power (FRAP)
To determine the antioxidant activity of the samples, the FRAP test was used (Benzie et al., 1996). The FRAP reagent was prepared freshly by mixing 25 ml of acetate buffer (300 mM, pH 3.6), 2.5 ml of tripyridyl-s-triazine solution (10 mM), and 2.5 ml of FeCl3 solution (20 mM). The reagent was warmed to 37°C for 30 min, and then 50 μl of different concentrations of extracts (1000, 500, 250, 125, 62.5, and 31.25 μg/ml) were added to 950 μl of fresh FRAP reagent, and the absorbance was measured after an incubation time of 30 min at 37°C.
Antiproliferative activity
Cell culture
The adherent HepG2 human hepatoma cells (ATCC: HB-8065) were obtained from the American Type Culture Collection (ATCC, https://www.atcc.org/). The cells were cultured in Dulbecco’s Modified Eagle Medium (DMEM) supplemented with 10% (v/v) fetal calf serum and 0.1% (v/v) streptomycin. The cell culture was maintained at 37°C in a humidified incubator with 5% CO2 saturation.
Cell viability assay
To determine cell growth, the MTT test was used, which is based on the reduction of 3-(4,5-dimethylthiazol-2-yl)-2,5-diphenyltetrazolium bromide (MTT) by intact cells' mitochondrial dehydrogenase into an insoluble purple formazan product (Stockert et al., 2012). HepG2 cells were incubated in 96-well plates at a concentration of 5×103 cells per well. After a 24-h incubation period at 37°C, cells were treated for 24, 48, or 72 h with plant extracts at different concentrations (0.05–2.5 mg/ml). Control cells were incubated with serum-free medium (DMEM) or with DMSO (0.5 v/v%) at 37°C. Treated cells were incubated with 30 μl of MTT reagent for 2 h. A scanning multiwell spectrophotometer (Thermo Scientific Multiskan EX, Monza, Italy) set to 550 nm was used to measure the amount of formazan dye produced. The results were expressed as a percentage of cell viability relative to control (%). Experiments were performed in triplicate using at least two independent assays. The half-maximal inhibitory concentration (IC50) was also calculated from dose–response curves using Graph Pad Prism software (Graph Pad Software, Inc., La Jolla, CA, USA).
Tali TMapoptosis kit-annexin V Alexa Fluor 488 and propidium iodide
An early stage of apoptosis was determined using annexin V, as reported by Ota et al. (2006). An Alexa Fluor 488 annexin V/dead cell apoptosis kit (Molecular Probes, CA, USA) was used to evaluate apoptosis, which was defined as the translocation of phosphatidylserine to the cell surface. HepG2 cells (5×105 cells/well) were seeded in 12-well plates and treated with the MTT IC50 value of each extract for 24 h. The cells were then washed twice with PBS buffer, and stained with annexin V-FITC and propidium iodide (PI), and the results were assessed using flow cytometry (FACS Calibur, Becton Dickinson, USA).
Measurement of ROS production
The generation of intracellular reactive oxygen species (ROS) was evaluated using 2,7′-dichlorofluorescein diacetate (DCFH-DA) (Sigma Chemical Company, St. Louis, MO, USA) in both treated and control cells, as described by Chang et al. (2001). Cells were seeded at a density of 2×105 cells per well and treated with I. viscosa extracts at their respective IC50 values for 24 h. After incubation, cells were detached with trypsin-EDTA and washed with PBS. Control and treated cells were resuspended in 0.5 ml of PBS containing 10 M DCFH-DA and incubated with 4 mM H2O2 (as an inducer of ROS generation) for 30 min at 37°C. ROS production was measured using a luminescence spectrophotometer (Perkin-Elmer, MA).
Statistical analysis
The results were expressed as the mean ± standard deviation of three independent measurements. One-way analysis of variance, followed by Tukey's test, was performed using Graph Pad Prism 5.0 software (Graph Pad, San Diego, CA, USA). The data were considered statistically significant at P < 0.05.
In silico molecular docking study
The phenolic compounds of I. viscosa leaves identified by Kheyar-Kraouche et al. (2018) using liquid chromatography linked to photodiode array detection and electrospray ionization mass spectrometry (LC-DAD-ESIMS/MS) were utilized to determine the most active compounds that inhibit BCL-2 antiapoptotic protein. A total of 26 compounds, including 4-caffeoylquinic acid, chlorogenic acid, caffeic acid, caffeoylquinic acid, catechin, coumaroyl, delphinidin, dicaffeoylquinic acid, galloylquinic acid, (Epi), gallocatechin-gallate, genkwanin, naringenin, proanthocyanidin dimer, protocatechuic acid, quercetin rhamnoside, quercetin, banaxanthone e, rosmarinic acid, padmatin, cirsiliol, mangostin, spinacetin, rosmanol, paxanthone, caffeic acid phenethyl ester, medioresinol, hispidulin, and rhamnetin, were selected as ligands for the docking analysis. Venetoclax and Obatoclax, the first selective BCL-2 inhibitors for routine clinical use, were used as control ligands.
The 3D molecular structures of the ligands were obtained from PubChem and created using AutoDock Tools (Morris et al., 2009) by minimizing energy and adding hydrogen atoms and charges, as well as adjusting the number of active torsions. The results were saved in pdbqt format.
The crystal structure of human BCL-2 (PDB ID: 6QGK) was downloaded in pdb format from the RCSB Protein Data Bank (https://www.rcsb.org/). Using BIOVIA Discovery Studio v. 2021, water molecules, heteroatoms, and nonstandard ligands were removed from the macromolecules and saved in pdbqt format. AutoDock Vina software (Morris et al., 2009) was used to perform docking calculations. The target structures were docked with ligands, and the values of the grid boxes were determined through a series of trials and errors. For this investigation, the chosen grid box was (x 40% –0.698, y 40% –4.034, z 40% 20.268 at 0.375 Å spacing centered).
Multiple conformations for the ligand were generated in the docking procedure, and the final energy refinement of the ligand posture was performed. The docking score of the optimum pose into the target proteins was computed for all of the examined bioactive compounds.
Results
Extraction yield and phenolic compound contents
Table 1 shows the extraction yield, total phenols, flavonoids, and condensed tannins of I. viscosa leaves using different extraction solvents. The yields of bioactive compounds varied significantly depending on the solvent used. The highest extraction yields were obtained from the aqueous ethanol (70%) extract (33.98%), followed by water and aqueous ethyl acetate (70%) extracts (18.54 and 15.84%, respectively).
Table 1
Determination of extraction yield and phenolic compound contents in the I. viscosa plant extracts
The use of pure solvents resulted in the lowest levels of extraction yield (<10%). Moreover, the concentration of phenolic compounds was strongly affected by the extraction solvents used. Aqueous ethanol (70%) extract showed the highest concentration of total phenols (645.58 ± 8.77 mg CE/g). Similarly, this extract also exhibited a high level of flavonoids (150.50 ± 2.22 mg QE/g), although the highest level of flavonoids was obtained from the ethyl acetate (70%) extract (180.69 ± 1.54mg QE/g). Notably, pure chloroform extract contained a considerable level of condensed tannins (37.48 ± 0.67 mg TAE/g).
Antioxidant capacities of I. viscosa extracts
The antioxidant activity of I.viscosa leaf extracts was evaluated using ABTS radical scavenging activity and ferric reducing antioxidant power (FRAP) (Fig.1). The results showed that the antioxidant activity varied significantly depending on the extraction solvent used. Aqueous ethanol (70%)and water extracts had the best potential to scavenge ABTS, with values of 572.74 and 549.53 μmol TE/g extract, respectively. Notably, there was no significant difference (P < 0.05) between these two extracts. The lowest ABTS scavenging capacity was observed in the aqueous chloroform (70%) extract, with a value of 229.22 μmol TE/g extract.
Fig. 1
The trolox equivalent antioxidant capacities of various extractsof Inula viscosa using ABTS(A) and FRAP (B) methods; E1 – water, E2 – ethanol (100%), E3 – aqueous ethanol (70%), E4 – ethyl acetate (100%), E5 – aqueous ethyl acetate (70%), E6 – chlorofom (100%) and E7 – aqueous chloroform (70%)
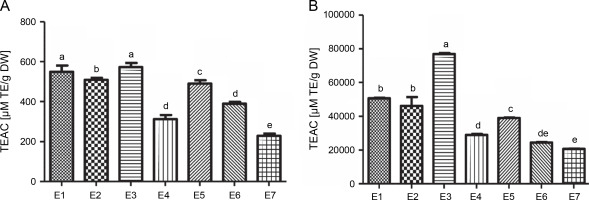
In contrast, the aqueous ethanol (70%) extract exhibited the highest reducing capacity with a value of 76 862.06 μM TE/g DW for the FRAP test, followed by water, ethanol (100%), aqueous ethyl acetate (70%), ethyl acetate (100%), chloroform (100%), and aqueous chloroform (70%)extracts, with values ranging between 20 729.88 and 50 660.92 μM TE/g DW.
Cell viability
To evaluate the cytotoxicity of I.viscosa leaf extracts on the proliferation of human liver cancer cell lines, HepG2 cells were treated with various concentrations of different extracts for 24, 48, or 72 h, and cellular viability was measured using the MTT assay to determine the IC50 values of I.viscosa extracts (Fig. 2). After 24 h of incubation, all extracts showed a significant (P < 0.05) cytotoxic effect against HepG2 cancer cells in a time-dependent and dose-dependent manner (Fig. 2A). As shown in Figure 2B, the aqueous ethanol (70%) extract exhibited the highest inhibitory effects, with an IC50 value of 1.67 mg/ml, followed by pure ethyl acetate and ethanol extracts. Pure chloroform (IC50 = 36.99 mg/ml) showed the lowest reduction in cell viability.
Fig. 2
Dose-dependent inhibition of human liver cancer cell (Hep G2) by I. viscosa extracts: (A) – cells were treated with DMSO vehicle or the indicated concentrations of I. viscosa extracts for 24 h; cell viability was determined using MTT assayand expressed as means ± SD of three independent experiments (n = 3), columns with different superscript letters are significantly different (P < 0.05); (B) – IC50 values of I.viscosa extracts were determined based on the dose–response curves; E1 – water, E2 – ethanol (100%), E3 – aqueous ethanol (70%), E4 – ethyl acetate (100%), E5 – aqueous ethyl acetate (70%), E6 – chloroform (100%) and E7 – aqueous chloroform (70%)
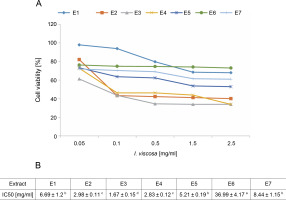
I. viscosa extracts induced apoptosis in HepG2 cells
Based on the MTT results, the aqueous ethanol (70%) and pure ethyl acetate extracts were chosen for further experiments to understand the mechanism of I. viscosa cytotoxicity. After 24 h of treatment, HepG2 cells were collected, and flow cytometry was utilized to quantify apoptosis using annexin-V Alexa fluor 488/PI staining (Fig. 3). The number of apoptotic cells significantly increased (P < 0.05) after extract treatment. The percentage of apoptotic cells in HepG2 cells treated with the aqueous ethanol and pure ethyl acetate extracts increased to 8 and 6%, respectively, in comparison to the control group (2% cells) without the extract (Fig. 3).
Fig. 3
I. viscosa extracts induced apoptotic cell death in human liver cancer cells (Hep G2) after treatment with IC50 for 24 h; values are expressed as mean ± SD of three independent experiments (n = 3); columns with different superscript letters are significantlydifferent (P < 0.05); CTL – untreated cells, E3 – aqueous ethanol, E4 – pure ethyl acetate
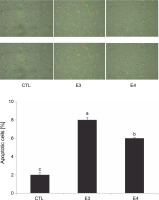
Effects of I. viscosa on ROS production in HepG2 cells
The effect of I. viscosa extract on intracellular ROS generation was studied by measuring changes in the fluorescence intensity of Cell-ROX Orange (Fig. 4).
Fig. 4
Effect of I.viscosa extracts on ROS intracellular production of Hep G2 after treatment with IC50 for 24 h; values are expressed as mean ± SD of three independent experiments (n =3); columns with different superscript letters are significantly different (P < 0.05); CTL – untreated cells, E3 – aqueous ethanol and E4 – pure ethyl acetate
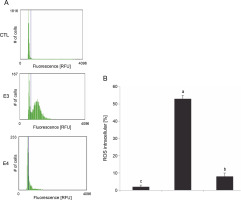
Based on the results presented in Figure 4, it can be observed that I. viscosa extracts induced a significant increase in intracellular ROS production in HepG2 cells in a solvent-dependent manner (P < 0.05). Notably, cells treated with the aqueous ethanol extract (IC50, 24h) exhibited the highest level of ROS production (53%) when compared to the control cells (2%) without extract (Fig. 4). Additionally, the level of ROS production was higher in cells treated with aqueous ethanol extract than in cells pretreated with pure ethyl acetate (8%).
Molecular docking studies
Overall, 26 phenolic compounds from I. viscosa and the standards (venetoclax and obatoclax) were dockedto the active site of BCL-2 (PDB ID: 6QGK). The ligands were classified according to their binding energy (kcal/mol) with the protein (Table 2). The interactions between antiapoptotic protein BCL-2 (PDB ID: 6QGK) and chemicals as well as the binding energies of the complexes were used to analyze the data.
Table 2
Phenolic compound docking results
All investigated ligands were compared with the standard molecules, obatoclax, and venetoclax, based on the interaction energy criteria (interaction energy: –7.7 and –7.8 kcal/mol, respectively). Among the 26 docked compounds (Table 2), Banaxanthone E (7.6 kcal/mol) and paxanthone (7.6 kcal/mol) exhibited good binding interaction with the antiapoptotic protein BCL-2. Therefore, these compounds were predicted to have the most stable molecular binding with BCL-2. Figure 5 illustrates the molecular interaction of Banaxanthone E and paxanthone in the active site of BCL-2. These compounds interact with the antiapoptotic protein BCL-2 through hydrogen and hydrophobic interactions. Paxanthone binds to the active site of BCL-2 through three hydrogen bonding interactions of free hydroxyl groups with Arg146 and Leu137 residues. Banaxanthone E, on the other hand, interacts with Val133, Leu119, Met115, Phe104, and Tyr108 residues through pi sigma, pi-alkyl, alkyl, and pi-sulfur interactions (Table 3).
Table 3
Interacting residues of BCL-2 with phenolic compounds from I. viscosa and the number of hydrophobic and hydrogen bonds
Discussion
In the last few decades, cancer treatment has primarily relied on cytotoxic chemotherapy and radiation therapy. However, the efficacy of these treatments has been hindered by their negative side effects and the development of resistance (Schirrmacher, 2019). To overcome these challenges, there is a need for new effective cancer therapies that are selective and have fewer side effects. Natural products have emerged as a promising source of anticancer medications, with many being more effective and less hazardous than traditional therapies (Rayan et al., 2017). Approximately 60% of cancer medications in use today are derived from natural compounds, many of which originate from plants (Newman and Cragg, 2020). Phenolic compounds, in particular, have demonstrated remarkable therapeutic and preventive properties against various forms of human cancer. They contain functional groups that enable them to exert multiple anticancer mechanisms, such as inducing apoptosis, autophagy, cell cycle arrest at different stages, and inhibiting telomerase (Islam et al., 2021; Rauf et al., 2022).
The leaves of I. viscosa are commonly used in traditional Algerian medicine to treat various ailments, including anti-helminthic, anti-inflammatory, antiseptic, antipyretic, anticancer, and diabetic therapy (Baba Aissa, 2000; Haoui et al., 2015; Ouahchia et al., 2020; Taïbi et al., 2020).
The extraction of active components is a crucial step in the production of herbal products, as it influences both the quality and quantity of the extracted components. Various factors can affect the extraction yield, such as the extraction method, solvent polarity, temperature, extraction time, sample particle size, pH, chemical nature of phytochemicals, and possible interferences from nonantioxidant compounds (Do et al., 2014; Pérez-Jiménez, et al., 2008). The extraction solvent, in particular, has a significant effect on the yield of extraction and antioxidant activity due to the existence of numerous antioxidant molecules with different chemical properties and polarity (Do et al., 2014; Metrouh-Amir et al., 2015; Sultana et al., 2009). It has been observed that the addition of water to organic solvents can increase the solubility of phenolic compounds by modulating the polarity of the organic solvent (Mohammedi et al., 2011).
In this study, the combination of organic solvents with water resulted in the highest extraction rate and phenolic compound content. The aqueous extract contained higher levels of total phenols and flavonoids, while the extracts obtained with pure solvents showed the highest amount of tannins. These findings are consistent with previous studies that have investigated the effect of solvents on extraction (Koofi et al., 2010; Metrouh-Amir et al., 2015). Metrouh-Amir et al. (2015) reported lower contents of total phenols, flavonoids, and condensed tannins using seven different solvents for the extraction of Matricaria pubescens compared to the results of the present study.
I. viscosa is a known source of various bioactive compounds, including phenolic acids, flavonoids, tannins, guaianolides, sesquiterpenes, sesquiterpene acids, triterpenoids, lactones, costic acid, and essential oils (Brahmi-Chendouh et al., 2019; Kheyar-Kraouche et al., 2018; Ozkan et al., 2019). Due to its high phenolic content, we investigated the antioxidant potential of I. viscosa extracts using two complementary assays (ABTS and FRAP). Our results showed that the aqueous extracts had significantly higher free radical scavenging activity than the pure solvent extracts. According to Babbar et al. (2014) and Temesgen et al. (2022), the differences in antioxidant capacity may be due to the quantity and quality of phenolic compounds present in each solvent.
Phenolic compounds exhibit antioxidant activity by donating hydrogen atoms to free radicals and scavenging other reactive species, such as OH•, NO2 •, N2O3, ONOOH, and HOCl. Additionally, some phenolics, especially diphenolsand polyphenols, can bind to transition metal ions, such as iron and copper, and react with oxygen, leading to forms that are ineffective at generating free radical reactions (Zin et al., 2004). The polarity of the solvent can also affect the antioxidant activity of the extract. Wakeel et al. (2019) and Abarca-Vargas et al. (2016) reported that solvent polarity can influence the extraction of a specific group of antioxidants, thereby affecting the antioxidant activity. Zhang et al. (2018) and Autor et al. (2022) demonstrated that highly polar organic solvents, such as water and alcohols (e.g., ethanol and methanol), are essential in improving phenolic compound extraction yield. Highly hydroxylated aglycone forms of phenolic compounds are soluble in high polar solvents, and the hydroxy groups in phenolic compounds contribute to their antioxidant activity (Kaczorová et al., 2021).
The second objective of this study was to assess the cytotoxicity of I. viscosa leaf extracts on the proliferation of human liver cancer cell lines (HepG2) and explore their cytotoxic mechanism. Previous studies have demonstrated the cytotoxic and anticancer activities of I. viscosa in various cancer cell lines. For example, Kheyar et al. (2022) found that the ethanolic extract of I. viscosa did not exhibit cytotoxicity on nondifferentiated Caco-2 cell lines, but showed growth inhibitory activity against human colon carcinoma HT29 cells. They concluded that I. viscosa extracts caused a significant decrease in cell viability in a dose-dependent manner.
In this study, we found that the aqueous ethanol (70%) and pure ethyl acetate extracts caused a significant reduction in cancer cell viability, which may be due to the presence of compounds with cytotoxic properties. Our extract’s phytochemical analysis (Kheyar-Kraouche et al., 2018) revealed the presence of various phenolic compounds, including chlorogenic acid derivatives, flavonoids, and sesquiterpenes. These compounds have been the focus of many studies, all of which have produced promising results and demonstrated significant inhibition of the proliferation of human cells from various types of cancer (Ayouaz et al., 2021; Huang et al., 2020; Macrì et al., 2020).
Previous studies have established the cytotoxicity potential of I. viscosa extracts on various cancer cell lines, with varying degrees of growth inhibition (Bar-Shalom et al., 2019; Belayachi et al., 2013; Merghoub et al., 2016; Sevgi et al., 2021; Talib et al., 2010; Virdis et al., 2020). For instance, Belayachi et al. (2013) observed a decrease in the proliferation of PC-3 prostate cancer cells in vitro after exposure to I. viscosa's dichloromethane extract. Additionally, Bar-Shalom et al. (2019) demonstrated the antiproliferative effect of I. viscosa’s hexanic extract on colorectal carcinoma cell lines in vitro. Recent study showed the effectiveness of I. viscosa ethanolic extract in reducing the proliferation of a human colorectal adenocarcinoma cell line (HT29) with an IC50 value of 62.39 ± 0.34 μg/ml (Kheyar et al., 2022).
Apoptosis is a crucial physiological mechanism that eliminates damaged or unwanted cells and is considered the preferred way to eliminate cancer cells (Kim et al., 2013; Xie et al., 2014). Defects in apoptosis enable cancer cells to become tumors and make them resistant to therapy (Hassan et al., 2014; Yu and Zhang, 2004). In this study, we examined the effects of I. viscosa extracts on apoptosis in the HepG2 cell line in vitro and intracellular ROS production to explore the mechanisms underlying I. viscosa's anticancer activity. Our Annexin V/PI flow cytometric assay showed that after 24 h of exposure to aqueous ethanol (70%) and pure ethyl acetate extracts, there was a significant increase in apoptosis to 8 and 6%, respectively. These results are consistent with previous studies that have demonstrated the anticancer properties of I. viscosa extracts. For example, Benbacer et al. (2012) reported that I. viscosa extracts had cytotoxic effects on cervical cancer cell lines by reducing proliferation and inducing apoptosis. Merghoub et al. (2016) showed that I. viscosa extracts promoted apoptosis in cervical cancer cells, and the active compounds overcame treatment resistance in neoplastic cells. Additionally, Bar-Shalom et al. (2019) found a proapoptotic effect of I. viscosa extracts on colorectal cancer in vitro and in vivo, with no adverse effects in animal models.
Several studies have shown that inducing intracellular ROS in cancer cells through bioactive compound therapy is a promising alternative in developing anticancer drugs. Increasing ROS levels through natural product therapy not only regulates the cell cycle butalso triggers apoptotic cell death by altering intracellular signaling molecules such as the Bcl-2 family and caspases (Kim et al., 2016). Our results demonstrate that I. viscosa extracts stimulate intracellular ROS generation and influence apoptosis in cancer cells. Talib et al. (2012) reported the ability of I. viscosa's phenolic compounds to induce apoptosis in MCF-7 cells, as evidenced by the presence of DNA fragmentation, nuclear condensation, and formation of apoptotic bodies in treated cancer cells. Indeed, the main phenolic components found in I. viscosa extracts were flavonoids, sesquiterpenes, chlorogenic acid, and its derivatives (Brahmi-Chendouh et al., 2019; Danino et al., 2009; Gökbulut, 2016; Kheyar-Kraouche et al., 2018; Sevgi et al., 2021). These molecules were found to have cytotoxicity and induce apoptosis or necrosis in various cancer cell lines, including human melanoma cell lines (Rozenblat et al., 2008), cultured oral KB epithelial cells (Chang et al., 2002), and osteosarcoma cells (Salzillo et al., 2021).
BCL-2 is a target for cancer therapy as it prevents programmed cell death induced by various stimuli, including hypoxia and oxidative stress (Frenzel et al., 2009). Therefore, developing novel Bcl-2 inhibitors from phytochemicals is of high interest. In this study, molecular docking was performed using AutoDock Vina software to examine the interaction between 26 phenolic compounds identified in I. viscosa’s ethanolic extract, as described by Kheyar-Kraouche et al. (2018), and BCL-2 (PDB ID: 6QGK). Through this study, we were able to define the amino acid residues involved in the binding interactions as well as the protein–ligand binding ener-
gies. BCL-2 is a well-known target in cancer research as it prevents programmed cell death induced by hypoxia and oxidative stress (Frenzel et al., 2009). Therefore, developing BCL-2 inhibitors from phytochemicals is of great interest. In this study, we performed molecular docking of 26 phenolic compounds identified in I. viscosa's ethanolic extract toward BCL-2 (PDB ID: 6QGK) using AutoDock Vina software. We were able to define the amino acid residues involved in the binding interactions and determine the protein–ligand binding energies. The study found that two xanthones, paxanthone and banaxanthone E, previously reported in I. viscosa extracts by Kheyar-Kraouche et al. (2018), hada good affinity for the examined antiapoptotic protein BCL-2, similar to obatoclax and venetoclax. Previous studies have reported that banaxanthone E showed cytotoxicity (Auranwiwat et al., 2016; Thanh et al., 2021) and exhibited antiproliferative activity by suppressing cell-cycle progression at the G2/M phase and activating the caspase pathway in human lung cancer cell lines (Thanh et al., 2021) and breast cancer cells (Nguyen et al., 2021). An interesting feature of this compound is that it binds through hydrophobic interactions with BCL-2's binding pocket. Several studies have shown that binding inhibitors with the hydrophobic groove of BCL-2 activate the apoptotic process (Souers et al., 2013; Verma et al., 2017; Vogler, 2014; Voss et al., 2010). Furthermore, Verma et al. (2017) demonstrated that hydrophobic interactions are critical for stable complex formation. Hydrogen bonds play a significant role in the binding modes and docking mechanism, and the key interactions between BCL-2's groove amino acids and paxanthone are hydrogen bonds. We used BIOVIA Discovery Studio v. 2021 to superimpose the redocked complex on BCL-2 (PDB ID: 6QGK), and our results showed that both this protein and banaxanthone E had a low root mean square deviation (RMSD) of 0.8 Å, comparable to the findings of Ismail et al. (2022a, 2022b), which suggested that docking accuracy increases with decreasing RMSD.
Conclusion
In summary, the results of this study suggest that I. viscosa has promising bioactive compounds with the potential as natural sources of antioxidants and anticancer agents. The choice of extraction solvent significantly influenced the extraction efficiency of phenolic compounds, which contributed to the antioxidant activity of the extracts. The aqueous ethanol (70%) and pure ethyl acetate extracts showed the most significant antiproliferative and apoptotic effects on HepG2 cells. Additionally, the molecular docking results indicated that paxanthone and banaxanthone E havea high affinity towards the antiapoptotic protein BCL-2 mainly through hydrophobic interactions. Further research on I. viscosa's potential anticancer properties should involve more cancer cell lines and animal studies. Furthermore, the isolation and characterization of bioactive compounds from I. viscosa should be the focus of future research to identify potential lead compounds for the development of natural anticancer agents.