Introduction
Gastric cancer (GC) represents a common cause of cancer death with high morbidity and mortality globally [1]. Recent reports have stated that an estimated 1.1 million new cases and approximately 0.8 million deaths from GC occurred in 2020 worldwide [2]. For gastric cancer patients, in addition to the traditional chemoradiotherapy and surgery, immunotherapy has emerged as a spectacular treatment strategy [1]. However, tumour immune evasion and resistance result in ineffective cancer immunotherapy [3]. Therefore, a better understanding of the immune responses to GC is urgent.
Among effector cells, NK cells are innate lymphocytes with cytotoxic activity against cancer cells and microbial infection by releasing cytokines and chemokines [4] and have shown great potential for immunotherapy [5]. NK cells and major stromal cells are independently prognostic and predict cancer chemotherapy benefit [6]. Meanwhile the percentage of tumour-infiltrating NK cells is decreased and associated with tumour progression and poor overall patient survival [7, 8] in terms of lower levels of activating receptor expression on circulating NK cells. Patients with a high level of NK infiltration are found to have a better prognosis than those with a low level of NK infiltration [9].
Abundant evidence indicates that the tumour micro- environment (TM) is a promising target for cancer therapy [10, 11]. Mesenchymal stem cells (MSCs) are a heterogenous stromal cell population with immunomodulatory, regenerative and differentiation properties which belong to stomal cells in the tumour environment (TME) [12]. There is an increasing body of evidence [13, 14] showing that tumour-associated MSCs have a strong impact on cancer initiation and progression. Moreover, our study has demonstrated that GCMSCs induce PD-L1 expression and can promote tumour development through paracrine signalling in GC [15, 16].
Meanwhile, MSCs possess systemic immunomodulatory and immunosuppressive properties and affect both adaptive and innate immune reactions directly or through the secretion of soluble molecules. Our previous study showed that GCMSCs impacted peripheral blood mononuclear cells via disruption of Treg/Th17 balance and PD-1 expression in CD4+ T cells to promote gastric cancer progression [17, 18]. For NK cells, it was reported that neuroblastoma cell lines with mesenchymal stromal cell phenotype have strong suppressive activity towards natural killer cells [19]. Although Guo et al. demonstrated that GCMSCs result in dysfunctional NK cells involved at least partially in the inhibition of mTOR signalling, the function and the mechanism of action of GCMSCs on NK cells are still not well unexplored [20].
It is known that metabolism affects NK cell differentiation and effector functions including the production of interferon γ (IFN-γ) and the lysis of target cells [21, 22]. Immunometabolism represents an intriguing target to improve immunotherapies [23]. Thus, the effect of GCMSCs on NK cells is considered in relation to the metabolism. Fructose-1,6-bisphosphatase (FBP1), a gluconeogenesis regulatory enzyme, mainly facilitates gluconeogenesis and inhibits glycolysis [24]. Also, increasing studies have suggested that FBP1 exerts tumour suppressor function in many types of human cancers in a manner dependent or independent of its enzymatic activity [25], e.g., hepatocellular carcinoma, pancreatic cancer, renal carcinoma. However, the investigation of FBP1 function in immunity cells is limited. Remarkably, Cong et al. found that aberrant FBP1 expression in NK cells reduced their dysfunction by inhibiting glycolysis and viability during lung cancer progression [26]. Thus, we propose a hypothesis that GCMSCs from TME may affect FBP1 expression in NK cells resulting in dysfunction and immune evasion in cancer.
Material and methods
Cell lines and cell culture
NK92 was kindly provided from Binzhou Medical University in Shandong and cultured in SuperCulture L-500 (SL51903, DAKEWE) containing 10% foetal bovine serum (FBS), interleukin (IL-2; 100 IU/ml, PeproTech). The human GC cell line HGC-27 was obtained from the Chinese Academy of Sciences Type Culture Collection Committee Cell Bank (Shanghai, China). HGC-27 cells were cultured in RPMI-1640 with 10% FBS (Biological Industries). Surgical gastric cancer tissues were derived from four gastric cancer patients at the Department of Surgery of the Aliated Hospital of Jiangsu University. Informed consent was obtained from all patients. The study was approved by the local Ethics Committee of the Aliated Hospital of Jiangsu University, China. GCMSCs were isolated from human GC tissues and identified in accordance with a previously described method [20]. All cells were maintained at 37°C in humid air with 5% CO2.
Osteogenic and adipogenic differentiation
GCMSCs were cultured in Dulbecco’s modified eagle medium (DMEM) at density of 2 × 104 cells per well in a 6-well plate, using differentiation inducing medium (Cyagen Biosciences, USA) to detect the ability of osteogenic and adipogenic differentiation of GCMSCs. At the end of induction, the plates were stained with Alizarin red to detect calcification and stained with fresh Oil Red-O solution to identify the presence of lipid droplets.
Conditioned medium of GCMSCs (GCMSCs-CM) preparation
GCMSCs reached 80% confluence, then were incubated with fresh MEM-ALPHA supplemented with 10% FBS for 48 h. Subsequently, the supernatant was collected, centrifuged at 1,000 g for 5 min to remove cells and filtered through a 0.22 µm membrane (Millipore, Germany). In the series of experiments, the supernatant was premixed with SuperCulture L-500 with 10% FBS at a volume ratio of 1 : 1, which was GCMSCs-conditioned medium (GCMSCs-CM). The controls were cultured using two mixed nutrient solutions (SuperCulture L-500 and MEM-ALPHA medium) at a ratio of 1 : 1.
NK cell isolation
Peripheral blood mononuclear cells (PBMCs) from healthy donors were separated through human lymphocyte separation medium density (Dakewe) gradient centrifugation. CD56+ NK cells were obtained after conjugated CD56 antibody positive selection with magnetic beads (Miltenyi Biotec). Freshly enriched NK cells were cultured in L500 medium and used in subsequent experiments.
Apoptosis analysis
Apoptosis of NK92 cells treated with GCMSC-CM for 48 h was detected using Annexin-V-FITC Apoptosis Detection Kit I (BD Pharmingen, Germany) according to the manufacturer’s instructions. In short, cells were resuspended in 1× binding buffer at a concentration of 1 × 106 cells/ml and 5 µl of annexin V-FITC and PI were added to each tube. Apoptosis was analysed by flow cytometry (Beckman Coulter) immediately and the apoptotic cells were annexin-V-FITC-positive.
Cell proliferation assay
Cell proliferation of the experimental and control groups was investigated by use of a Cell Counting Kit-8 (CCK8) assay (Beyotime Biotechnology). Briefly, NK cells were seeded in 96-well cell plates with 1 × 105 cells/well. Then, 10 µl of CCK-8 reagents were added to each well for 4-h incubation at 37°C. Immediately after, the absorbance was measured at 450 nm with an automated plate reader (BioTek, Winooski, VT, USA).
Cytotoxicity assay
To determine cytotoxicity, the CytoTox96 Non-Radioactive Cytotoxicity Assay kit (Promega, USA) was used to detect the level of lactate dehydrogenase (LDH) according to the manufacturer’s instructions. HGC-27 cells were seeded in a 96-well plate (5000 cells/well) in three to four replicates, then NK92 cells treated with the pre-mixed GCMSCS-CM for 48 h were added at Effector to Target (E : T) ratios of 10 : 1, 5 : 1, 1 : 1 at 37oC in a 5% CO2 incubator for 6 h. The supernatant was then collected and used for measuring the amount of LDH which was determined by measuring absorbance at 490 nm with a microplate reader (PT-3502C, Beijing). The killing effect of NK92 cells against HGC-27 cells was calculated by the following formula: Cytotoxicity = (Experimental – Effector spontaneous – Target spontaneous)/(Target maximum – Target spontaneous) × 100%.
Immunoblotting
Total proteins of NK92 cells after being treated with conditioned medium of GCMSCs for 48 h were extracted using the RIPA lysis buer (Invitrogen, Carlsbad, CA, USA) and separated by 12% SDS-PAGE, then transferred to PVDF (Millipore, Billerica, MA, USA). The membranes blocked with 5% non-fat milk were incubated with primary rabbit antibodies against FBP1 (dilution, 1 : 1,000; ab109732, Abcam, UK) overnight at 4oC, and then incubated with goat anti-rabbit (dilution, 1 : 2,000; CW0103, CWBIO, Beijing, China) secondary antibodies for 60 min at 37oC. After incubation, the signals were detected with ECL reagents (Millipore, Germany). β-actin was used as the loading control.
Glucose uptake and lactate production assay
A clinical chemistry analyser (ABBOTT, ci8200, USA) was utilized to detect the supernatant glucose concentrations which evaluated the eect of GCMSCS-CM on the glucose utilization in NK92 cells. In short, NK92 cells were treated with L-500 + DMSO, GCMSCs-CM + DMSO, GCMSCs-CM + MB05032 for 48 h, then seeded in 24-well plates (Corning Inc., Corning, NY, USA), incubated for 10 h in L-500 and the cell culture supernatant was collected. Lactate levels in supernatant were determined using a lactic acid test kit (KeyGEN BioTECH, Nanjing, China) according to the instructions of the manufacturer. Relative levels of lactate production and glucose uptake were normalized to the control group.
Flow cytometry and antibodies
A single cell suspension was prepared and incubated with the monoclonal antibody against CD107a-APC (328620, Biolegend), CD19, CD34, CD45, CD29, CD90 and CD105 (BD Biosciences, USA) conjugated with phycoerythrin (PE) or fluorescein isothiocyanate (FITC) for 30 min at 4°C in the dark. After washing, the labelled cells were suspended in PBS and were analysed using a flow cytometer (Beckman Coulter, CytoFLEX, USA) to detect NK92 cells degranulation capacity. To evaluate intracellular perforin or FBP1 expression, cells were fixed and permeabilized with eBioscience FoxP3 fixation buffer according to the manufacturer’s instructions, then analysed by flow cytometry using fluorescence antibody perforin-APC (353311, Biolegend) or antibody FBP1, which was subsequently incubated with secondary antibody conjugated with DyLight 649 (Abbkine). The data were analysed using FlowJo software. Isotype-matched antibodies were used in the control group.
Statistics
Each experiment was repeated independently in triplicate at least. Data were expressed as means ± SD and analysed using Graph-Pad Prism software 8.0.2 (GraphPad Software, USA). Statistical analysis was performed by the independent t-test between two groups or one-way analysis of variance (ANOVA). A p value < 0.05 was considered to be significant.
Results
Characterization of MSCs isolated from GC patients
GCMSCs isolated from the surgical gastric cancer tissues were spindle-shaped (Fig. 1A). The experiments showed that GCMSCs successfully underwent differentiation to adipocytes (Fig. 1B) or osteocytes (Fig. 1C) in vitro in response to appropriate stimulation. Flow cytometry analysis showed that GCMSCs were negative for CD19, CD34 and CD45 (Fig. 1E), but positive for CD29, CD90 and CD105 (Fig. 1E), which displayed the characteristic surface markers of GCMSCs.
Fig. 1
Morphological and phenotypic characteristics of GCMSCs. A) Representative morphological image of GCMSCs at the third passage (40×). B) Adipogenic dierentiation (Oil red O, 200×). C) Alkaline phosphatase staining detection for osteogenic differentiation (200×). D, E) Flow cytometric analysis of the surface markers (CD19, CD29, CD34, CD45, CD90, and CD105) on GCMSCs
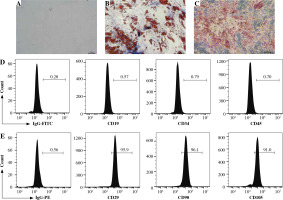
GCMSCs signicantly attenuated degranulation (CD107a), perforin production, and cytotoxicity in NK cells
To investigate the effect of GCMSCs on NK cells, proliferation and apoptosis of NK92 cells treated with GCMSCs-CM or not were evaluated. It was found that there was no significant difference in proliferation (Fig. 2A) or apoptosis (Fig. 2B) of GCMSC-CM-primed NK92 cells. Furthermore, CD107 expression of NK92 cells was impaired with GCMSCs-CM treatment, suggesting the decreasing degranulation capacity (Fig. 2C, D). We next investigated the functional capacities of NK92 cells and found that the frequencies of perforin positive cells (Fig. 2E, F) measured by flow cytometry were significantly lower in the control group compared with the GCMSCs-CM treatment group. Also, the effect of GCMSCs on NK92 cells was investigated in direct co-culture conditions and decreased CD107a and perforin expression in the presence of GCMSCs was found (Supplementary Fig. 1A-D). We were more interested in investigating the impact of components of the supernatant on NK cells; thus GCMSC-CM were used for further experiments. Also, it was found that CD107 expression (Fig. 3A, B) of GCMSC-CM-primed enriched NK cells in PBMC was attenuated; nevertheless perforin showed no significant difference (Fig. 3C, D). Several studies have reported that endogenous and exogenous ROS induce NK cells in a hypofunctional state [27]. Notably, we found that the intracellular ROS level of NK92 cells in the GCMSCs-CM increased significantly, in accordance with their impaired immunity function (Fig. 3E). To further investigate the eect of GCMSCs on NK cell antitumour activity, NK-mediated killing of gastric cancer cells HGC-27 was examined. It was found that NK92 cells treated without GCMSCs-CM co-cultured with HGC-27 cells resulted in more cancer cell death. It was suggested that GCMSCs attenuated cytotoxicity of NK cells (Fig. 3F). Altogether, these outcomes show that GCMSCs have an important effect on NK cells and induce NK92 cell dysfunction and low antitumour activity.
Fig. 2
GCMSCs-CM suppressed NK cell degranulation, perforin production and cytotoxicity. A) Proliferation of NK92 cells treated with GCMSCs-CM was detected by CCK-8 assays. B) After co-culture with control CM, GCMSCs-CM for 48 h, NK92 cells were collected and stained with annexin V/PI (n = 3). NK92 cells treated with control CM and GCMSCs-CM for 48 h were analysed through ow cytometry for CD107a (C) and perforin (E). Quantication of the per- centages of CD107a positive (D) and perforin positive (F) cells are shown. Data are shown as mean ± SEM. A paired t test was used for comparisons. *p < 0.05, ns, p > 0.05
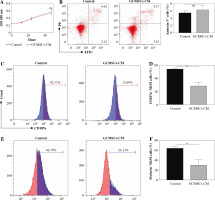
Fig. 3
GCMSCs-CM attenuated CD107a expression of enriched NK cells (CD56+). GCMSCs-CM-primed enriched NK cells were assessed through ow cytometry for CD107a (A) and perforin (B). C) Quantication of CD107a expression in enriched NK cells. D) Quantication of perforin expression in enriched NK cells. E) ROS levels in NK92 cells cultured with or without GCMSCs-CM were detected using the ROS assay kit. F) GCMSCs-CM-primed or unprimed NK92 cells were then co-cultured with HGC-27 cells for 6 h at 10 : 1, 5 : 1 or 1 : 1 effector : target ratios. Data are shown as mean ± SEM. A paired t test was used for comparisons. *p < 0.05, ns, p > 0.05
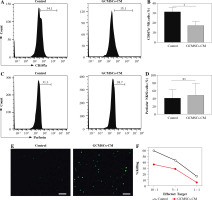
GCMSCs decreased glucose metabolism and promoted FBP1 expression in NK cells
Given that glucose metabolism is required for NK cell anti-tumour activity, we investigated whether NK92 cells treated by GCMSCs have altered metabolism. We found that GCMSCs-CM reduced glucose consumption capacity (Fig. 4A), indicating impaired metabolism ability. Lactate production level of treated NK92 cells was lower relative to NK92 cells (Fig. 4B). These results demonstrate that dysfunction of NK cells affected by GCMSCs corresponds with an impairment in glycolysis of NK cells. It is reported that FBP1 acts as a key molecule resulting in disabled NK cells in the tumour microenvironment by weakening their glycolytic metabolism [26]. Thus, we hypothesize that GCMSCs affect the metabolism of NK cells by upregulating FBP1 expression, inducing NK cell disfunction. Consequently, the increased expression of FBP1 in NK92 cells treated with GCMSCs or GCMSCs-CM supports the hypothesis (Fig. 4C, D).
Fig. 4
GCMSCs-CM decrease glucose metabolism and promote FBP1 expression of NK92 cells. A) Glucose uptake in NK92 cells treated with the GCMSCs-CM or control medium. B) Lactate production in NK92 cells treated with the GCMSCs-CM or control medium. C) Immunoblotting of FBP1 expression in NK92 cells treated with control medium or GCMSCs-CM for 48 h. D) Quantitative statistics of FBP1 expressed in different groups. Data are shown as mean ± SEM
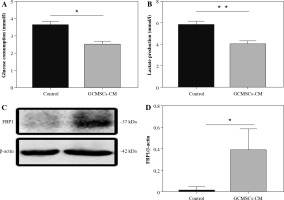
FBP1 inhibition restored the function of NK cells
Given the observation that NK92 cells showed a higher level of FBP1 expression and lower level of glucose metabolism when treated by GCMSCs-CM, we supposed that NK cell function could be mediated by FBP1 through glycolysis inhibition. Firstly, MB05032, an inhibitor of FBP1 which was verified as effective through flow cytometry (Fig. 5A, B), or DMSO (control) was added with GCMSCs-CM. As expected, GCMSCs-CM added with inhibitor did not affect the NK cell glucose consumption capacity (Fig. 5C) or lactate production level (Fig. 5D), showing that FBP1 inhibited glycolysis in NK cells directly. Then, the effect of FBP1 on the effector function was explored. It was found that FBP1 inhibition increased the NK92 cell degranulation capacity (Fig. 5E, F) and perforin production (Fig. 5G, H) compared with the GCMSCs-CM group. In addition, NK92 cell cytotoxicity was restored when FBP1 inhibitor was added (Fig. 5I). Furthermore, these results suggest that FBP1 plays an important role in NK cell functional fate.
Fig. 5
Inhibition of FBP1 increased by GCMSCs-CM restores the function of NK cells. A) NK92 cells treated with DMSO, GCMSCs-CM, and GCMSCs-CM + MB05032 (5 μM) for 48 h were analysed through ow cytometry for FBP1. B) Quantication of the percentages of FBP1 positive cells is shown. C) Glucose uptake in NK92 cells treated with the DMSO group, GCMSCs-CM group and GCMSCs-CM + MB05032 group. D) Lactate production in NK92 cells treated with the DMSO group, GCMSCs-CM group and GCMSCs-CM + MB05032 group. NK92 cells treated with DMSO, GCMSCs-CM and GCMSCs-CM + MB05032 for 48 h were analysed through ow cytometry for CD107a (E) and perforin (F) GCMSCs-CM and GCMSCs-CM + MB05032 for 48 h were analysed through ow cytometry for CD107a (E) and perforin (F). Quantication of the percentages of CD107a positive cells (G) and perforin positive (I) is shown. (H) GCMSCs-CM-primed, GCMSCs-CM-primed + MB05032 or unprimed NK92 were then co-cultured with HGC-27 cells for 6 hours at 10 : 1. n = 3, *p < 0.05, **p < 0.01
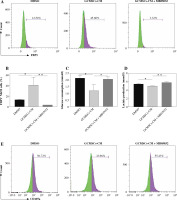
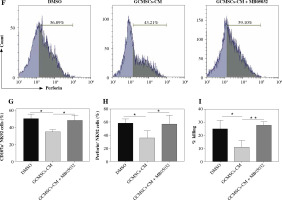
Discussion
Tumours develop within a mixed TME of epithelial and MSCs, vascular and lymphatic vessels, inflammatory and infiltrating immune cells [28]. A growing body of evidence suggests that the immunosuppressive TME is involved in tumour initiation, progression and immune evasion [29]. MSCs are known to tend to different tissues and play an essential role in TME [30]. On the other hand, NK cells are unique cytotoxic innate lymphocytes to target cancer cells without antigen specificity different from T and B cells, which makes them an excellent candidate for usage against solid tumours [31]. During the progression of GC, the number and activity of NK cells, which are correlated with clinical stage, lymphatic and vascular invasion, lymph node metastases, and prognosis in GC patients, are attenuated [32]. However, it is still unclear whether tumour resident MSCs in GC suppress anti-tumour responses of NK cells. In the current study, we provide evidence that GCMSCs, as important resident stromal cells in the GC microenvironment, could suppress anti-tumour immune responses of NK cells by attenuating CD107a and cytolytic molecules’ expression.
Increasing literature has highlighted that change in cellular metabolism is tightly linked to the functional fate of immune cells [33-35]. Previously, Slattery et al. observed that in human metastatic breast cancer, peripheral blood NK cells from patients had clear metabolic deficits including reduced glycolysis and oxidative phosphorylation (OxPhos), resulting in reduced INF-γ production and cytotoxicity [5]. If NK cells cannot keep up elevated rates of glycolysis or OxPhos, they will have impaired effector responses [36]. Consistently, we found that GCMSCs affect the NK cell glucose consumption capacity and lactate production level. That may explain why NK cells present an impaired state with reduced CD107, perforin expression and antitumour activity when they are treated with GCMSCs-CM. Further, we observed that GCMSCs resulted in elevated levels of FBP1 in NK cells. It was well documented that disabled NK cell glycolytic metabolism was weakened in the TME and FBP1 acts as an important molecule in the process [26]. In our study, it was also found that GCMSC-CM-primed NK92 cell dysfunction was reversed when using FBP1 inhibitor. Thus, FBP1 provided a theoretical basis for the development of new therapies for gastric cancer in the future.
There has been great interest in developing immunotherapy for cancer treatment in recent years. Intriguingly, some patients will benefit from combinations, e.g., co-targeting metabolic changes and PD-L1 [37] or CTLA-4 [38] rather than from monotherapy. Poznanski et al. cultured the expanded NK cells using IL-21-expressing feeder cells which have metabolic adaptations and can harbour features to better sustain function in the TME [39]. Simultaneously, it was reported that efficient immunotherapies could be developed when metabolism of chimeric antigen receptor NK cells was engineered [40]. Immunometabolism represents an attractive target; thus, it is important to understand how GCMSCs affect NK cell metabolism and function. While several experimental proofs have suggested that GCMSCs could disable NK cell function and metabolism by upregulating the expression of FBP1, there are still many questions unaddressed so far. It is worth investigating whether the molecule or exosomes from GCMSCs-CM attenuate NK cells and related mechanisms.
Overall, this study shows that NK cell function and metabolism are suppressed by GCMSCs. This impairment was mediated by FBP1 and our results provide new evidence for anti-GC immunotherapy by targeting the expression of FBP1.