Introduction
Dendrobium is the second largest genus in the Orchidaceae family, which consists of more than 25 000 species worldwide that belong to 880 genera (Niu et al., 2017). Commonly known as “Shih Hu” in traditional Chinese medicine, Dendrobium has been used for stomach nourishment, to treat flu, as a tonic, and to increase the production of body fluid (Shiau et al., 2005; Li et al., 2021). Various chemical compounds can be found in Dendrobium orchids, such as dendrobine and the previously unknown alkaloid nobiline. The main components of diverse compounds found in Dendrobium are alkaloids, aromatics, sesquiterpenoids, and polysaccharides (Robustelli della Cuna et al., 2021).
In vitro culture is the best method to propagate orchids as it can mass-produce plantlets that maintain good qualities and genetic stability of the plant (Cardoso et al., 2020). Protocorm-like bodies (PLBs) are a unique structure of orchids, and they are the initial structure formed in embryo development during seed germination (Teixeira da Silva et al., 2015; Santos et al., 2016). PLBs are often used by researchers for studies as they can proliferate easily and develop directly into shoots and roots (Teixeira da Silva et al., 2015). All parts of the orchid plant can be used as explants to produce PLBs, such as leaves, roots, stem nodes, apical buds, flower stalk, and seed (Zhao et al., 2008; Malik et al., 2021).
The culture vessel conditions play an important role in maintaining the growth of the orchid tissue cultures (Chen, 2003; Vasudevan and Van Staden, 2011). Different types and sizes of culture vessels affect the culture production efficiency (Islam et al., 2005). The types of the culture vessel can have an impact on light penetration, ventilation rate, and gaseous exchange during the production of plantlets (Patel et al., 2016). The size of the culture vessel can also affect plantlets’ development. A culture vessel with a larger volume tends to increase the production of shoots and roots because of the efficient dispersal of ethylene gas inside the vessel in addition to allowing for more accessible oxygen. The present study is the first study on the size of culture vessels used for growing Dendrobium Sabin Blue orchid.
Therefore, this study aimed to investigate the impact of using plastic and glass culture vessels on the proliferation and growth of PLBs in terms of growth index based on various biochemical analyses and to examine morphological and anatomical aspects of PLBs using various treatments.
Materials and methods
Plant material
Sixty-day-old PLBs of Dendrobium Sabin Blue orchid (1–2 mm) were obtained from the Plant Biotechnology Laboratory, School of Biological Sciences, Universiti Sains Malaysia, Penang. The PLBs were grown and maintained in half-strength Murashige and Skoog (MS) (1962) medium supplemented with 1 mg/l benzylaminopurine (BAP) under a 16 h light/8 h dark photoperiod with cool fluorescent light at 25 ± 2°C. Two types of culture vessels, namely plastic and glass vessels, were used in this study. The plastic culture vessels were of four sizes: 120, 240, 350, and 470 ml, while the glass culture vessels were of two sizes: 280 and 530 ml. The plastic culture vessels were manufactured by Ecoplastic Sdn. Bhd. (Malaysia) and purchased at Tesco Sungai Dua, Penang, while the glass vessels were purchased from JG Containers Malaysia Sdn. Bhd.
Culture of PLBs
Sterile plastic and glass culture vessels were used to culture seven PLBs individually. In each vessel, 0.145 g fresh weight of the seven PLBs was used as the initial mass. Eight replicates were made using both types of culture vessels. Therefore, the total number of samples was 112. PLBs were cultured in half-strength MS medium supplemented with 1 mg/l BAP and solidified with 3 g/l Gelrite. The pH of the medium was adjusted between 5.7 to 5.8 before autoclaving at 121°C for 20 min under 1.05 kg/cm2 pressure. Each vessel contained 30 ml of culture medium. These cultures were maintained in a 16 h light/8 h dark photoperiod under cool fluorescent light at 25 ± 2°C with 44 ± 9 μE/m2 ∙ s1 light intensity. After 11 weeks of culture, the growth index of the PLBs from culture vessel of each size was calculated using the following formula.
Biochemical analyses
Carbohydrate analysis
To determine carbohydrate content, 0.1 g of chopped PLBs from each treatment was used. Samples were transferred to a 25 ml glass test tube to which 15 ml of 80% (v/v) ethanol was added subsequently. The tubes were then incubated in a 60°C water bath for 30 min. After incubation, the samples were filtered through a Whatman No. 1 filter paper. Next, 4 ml anthrone solution was added to the extracted samples. The extracted samples were then incubated in a 95°C water bath for 30 min and allowed to cool down. The absorbance was recorded at 630 nm for carbohydrate determination by using a spectrophotometer (UV mini-1240, Shimadzu, Japan).
Chlorophyll, carotenoid, and porphyrin analyses
To determine the content of chlorophyll, porphyrin, and carotenoid, 0.1 g of PLBs was collected from each treatment and grounded with 1 g of calcium carbonate (CaCO3). Next, 25 ml of 80% acetone was added to the powder and mixed evenly. The mixtures were filtered through a Whatman No. 1 filter paper, and the filtrate was collected to determine the content of chlorophyll, porphyrin, and carotenoid. The absorbance was recorded at 665 and 649 nm for chlorophyll determination. Total chlorophyll, carotenoid, and porphyrin were determined according to Harborne (1973) by using the following equations:
The absorbance was measured at 440 nm for carotenoid determination, and the carotenoid content was calculated using the following equation:
The total porphyrin was calculated by equating the sum of protoporphyrin, Mg-protoporphyrin, and protochlorophyllide. To determine total porphyrin, the absorbances were measured at 575, 590, and 628 nm, respectively. Protoporphyrin, Mg-protoporphyrin, and protochlorophyllide were determined using the following equations:
Proline analysis
Proline analysis was performed according to Bates et al. (1973). Ninhydrin (1.25 g) was added to 60 ml of glacial acetic acid and 40 ml of 6M phosphoric acid. The mixture was agitated until dissolved and stored in a refrigerator for 24 h to stabilize the mixture. Chopped PLBs weighing 0.5 g were added to 15 ml of ethanol and immersed in a 60°C water bath for 30 min. The sample mixture was filtered with a filter paper, and the supernatant was collected. Then, 2 ml ninhydrin acid and 2 ml glacial acetic acid were added together to the filtrate. The mixture was then placed in a 100°C water bath for 1 h and cooled rapidly using ice water to immediately stop further reaction. Next, 4 ml of toluene was added to the mixture and shaken vigorously for 15 s. The absorbance of the mixture was recorded at 520 nm wavelength.
Histological analysis
Histological analysis was performed by fixing the PLBs in formaldehyde acetic acid (FAA). After 1 week, FAA was removed, and the PLBs were serially dehydrated with different concentrations of tertiary butyl alcohol (TBA) solutions (Gnasekaran et al., 2014), embedded in paraffin wax, and sectioned at 10 μm thickness using a microtome (Leica, United States). The samples were then stained with safranin and fast green (Hong et al., 2008). Finally, the sample slides were observed and photographed using a light microscope equipped with a video camera (Olympus BX5, Japan).
Scanning electron microscopy
PLBs were analyzed by scanning electron microscopy (SEM) based on the freeze-drying method. The freeze-drying method involved vapor fixing of the samples with 1% osmium tetroxide for 1 h, followed by freeze-drying (Emitech K 750 X) of the samples in liquid nitrogen (–210°C) slush and coating with 5–10 nm of gold sputter (Polaron SC515 sputter Coater, UK). The analysis was conducted using a scanning electron microscope (Leo Supra 50VP Field Emission SEM, Germany), and all images were processed digitally using Oxford INCA 400 energy dispersive X-ray microanalysis system software.
Statistical analysis
The experiments were performed under a completely randomized design (CRD). All data were analyzed by one-way analysis of variance (ANOVA) using Statistical Package for Social Sciences (SPSS) Software version 22.0. Comparisons of mean and standard errors were performed by Duncan’s multiple range tests at P ≤ 0.05 significance level.
Results
Growth index of PLBs
The growth index of PLBs in the plastic vessel gradually increased from 1.367 in the smallest 120 ml vessel to 7.36 in the largest 470 ml vessel (Fig. 1). PLBs in the other two plastic vessels, 240 ml and 350 ml, exhibited similar growth indices of 4.374 and 5.09, respectively. In glass vessels, the growth index showed no significant difference between the two vessel types of 280 ml and 530 ml, reaching 7.123 and 6.52, respectively (Fig. 2). The highest number of PLBs was obtained using the 470 ml plastic vessel (Fig. 3). PLBs grew larger in 120 ml plastic vessels but did not form shoots or roots, whereas PLBs in 240, 350, and 470 ml plastic vessels developed shoots. In glass culture vessels, complete plantlets with shoots and roots were obtained (Fig. 4).
Fig. 1
Effect of different sizes of plastic vessels on the growth of PLBs after 11 weeks of culture; data were analyzed by one-way ANOVA; vessels assigned with the same letter show no significant difference based on Duncan’s multiple range test (P ≤ 0.05)
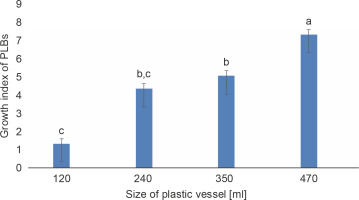
Fig. 2
Effect of different sizes of glass vessels on the growth of PLBs after 11 weeks of culture; data were analyzed by one-way ANOVA; vessels assigned with the same letter show no significant difference based on Duncan’s Multiple Test (P ≤ 0.05)
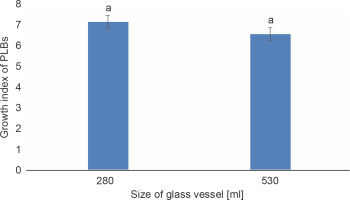
Biochemical analyses
Carbohydrate analysis
The carbohydrate content of PLBs was significantly influenced by different types of culture vessels (Table 1). Among plastic vessels, PLBs growing in 470 ml plastic vessels produced the highest carbohydrate content (2.671 mg/g), whereas PLBs growing in 120 ml plastic vessels had the lowest carbohydrate content (1.941mg/g). The carbohydrate content of PLBs was 2.078 mg/g fresh weight in the 530 ml glass culture vessel. PLBs growing in the 280 ml glass culture vessel showed carbohydrate content of 1.449 mg/g fresh weight. Based on the histological observation, a higher amount of starch granules was present in PLBs cultured in the 350 ml plastic vessel than in PLBs cultured in the 240 ml vessel which showed only a small amount of starch granules (Fig. 5).
Table 1
Effect of different sizes of plastic and glass vessels on the carbohydrate and proline content in the PLBs after 11 weeks of culture
Proline analysis
The size of culture vessels had a significant impact on proline content in PLBs (Table 1). The highest amount of proline (24.38 nmol/mg fresh weight) was observed in PLBs growing in a 120 ml small plastic culture vessel. The proline content of PLBs growing in the 240 ml and 350 ml plastic culture vessels decreased dramatically to 6.789 and 5.82 nmol/mg fresh weight, respectively. The lowest amount of proline at 5.395 nmol/mg fresh weight was obtained in PLBs growing in the 470 ml plastic culture vessel. PLBs cultured in 280 ml glass vessels showed a higher proline level of 27.016 nmol/mg fresh weight than those cultured in 530 ml glass vessels, with a proline content of 5.183 nmol/mg fresh weight.
Chlorophyll, carotenoid, and porphyrin analysis
The size of the culture vessels significantly affected the concentrations of chlorophyll a and b and total chlorophyll in PLBs (Table 2). The highest concentration of total chlorophyll (7.825 mg/g fresh weight) was obtained in PLBs cultured in the 470 ml plastic culture vessel, while the lowest concentration (1.256 mg/g fresh weight) was obtained in PLBs in 120 ml culture vessels. The highest chlorophyll a and chlorophyll b concentrations in PLBs grown in the largest 470 ml plastic vessel were 3.363 and 4.462 mg/g fresh weight, respectively. The lowest chlorophyll a (0.90 mg/g fresh weight) and chlorophyll b (0.356 mg/g fresh weight) concentrations were obtained in 120 ml cultured PLBs. The concentrations of chlorophyll in PLBs growing in glass culture vessels with larger and smaller sizes were significantly different. Total chlorophyll (2.069 mg/g fresh weight), chlorophyll a (1.105 mg/g fresh weight), and chlorophyll b (0.964 mg/g fresh weight) were observed to be the highest in the 530 ml glass vessel. In contrast, total chlorophyll (1.280 mg/g fresh weight), chlorophyll a (0.884 mg/g fresh weight), and chlorophyll b (0.379 mg/g fresh weight) were lower in the PLBs growing in the 280 ml glass vessel.
Table 2
Content of chlorophyll (a & b ) and total chlorophyll content in the PLBs grown in different sizes of plastic and glass vessels
The type and size of culture vessels showed a significant impact on the carotenoid content (Table 3). Carotenoid levels in the PLBs grown in the plastic vessels fluctuated as the vessel volume increased. The carotenoid content of PLBs in the 120 ml plastic vessel was 27.20 g/g fresh weight but decreased slightly to 23.727 g/g fresh weight in the 240 ml vessel. However, in the 350 ml plastic culture vessel, the growth of PLBs was boosted, and the concentration of carotenoid increased to 141.40 g/g fresh weight. In contrast, in the 470 ml plastic culture vessel, the carotenoid content of PLBs was decreased to 74.07 g/g fresh weight. PLBs cultured in 280 ml glass culture vessels showed higher carotenoid content (30.478 g/g fresh weight) than those grown in 530 ml glass vessels (21.605 g/g fresh weight).
Table 3
Content of carotenoid and porphyrin content in the PLBs grown in different sizes of plastic and glass vessels
Porphyrin content was also significantly influenced by the type and size of culture vessels (Table 3). PLBs grown in a 470 ml plastic vessel had the highest porphyrin content (40.521 g/g fresh weight), while those grown in the smallest vessel of 120 ml had 14.165 g/g fresh weight of porphyrin. PLBs grown in 240 ml vessels had slightly lower porphyrin concentration (8.096 g/g fresh weight) than those grown in 350 ml vessels (6.093 g/g fresh weight). The porphyrin content of PLBs in the glass jar decreased from 7.772 g/g fresh weight in the 280 ml vessel to 6.696 g/g fresh weight in the 530 ml vessel.
Histological analysis
PLBs from 120 ml plastic vessels had a compact tissue of dividing cells and a small number of starch granules (Fig. 5). PLBs grown in the 240 ml plastic culture vessel showed rapidly dividing cells and shoot formation. Starch granules were condensed with large vacuoles in the 350 ml vessel, whereas shoots, and starch granules were observed in PLBs grown in the 470 ml vessel. PLBs cultured in the 530 ml glass vessel had a high concentration of starch granules (Fig. 6), whereas those grown in the 280 ml glass vessel had a low concentration of starch granules.
SEM analysis
PLBs from the 120 ml plastic vessel had fewer stomatal pores (Fig. 7). In larger plastic culture vessels of 240 and 350 ml, PLBs showed a higher number of more developed and highly distributed stomatal pores. Rhizoid formation was observed on PLBs growing in plastic vessels (350 ml) and glass vessels (280 ml) (Fig. 8). PLBs growing in the 530 ml glass vessel exhibited partially grown stomatal holes (Fig. 9).
Fig. 7
SEM analysis of 11-week-old PLBs cultured in different types and sizes of vessels: A) 120 ml plastic vessel, B) 240 mlplastic vessel, C) 280 ml glass vessel, D) 350 ml plastic vessel, E) 470 ml plastic vessel, F) 530 ml glass vessel
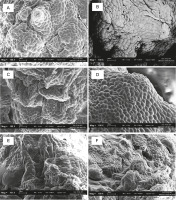
Discussion
In general, it is essential to optimize in vitro culture conditions for growing cultures. Explants when grown in vitro face many stress conditions due to the availability of gases and nutrients for the growth of cultures. The culture vessel size and type largely influence plant growth and pigment concentration (Martins et al., 2015). In the current experiment, we analyzed and reported the impact of large-sized plastic and glass culture vessels on the growth of PLBs and pigment concentration.
This study found that the different sizes and types of culture vessels used for the experiment affected the fresh weight of PLBs. The main highlight of this experiment was that, following the use of large-sized culture vessels, the total yield of PLBs increased, resulting in increased concentrations of pigments such as chlorophyll, carotenoids, and porphyrins. It is known that different vessel sizes have a significant impact on the microenvironment inside the vessel (Martins et al., 2015), especially on the concentrations of oxygen and carbon dioxide, along with other volatiles such as ethylene in the air inside the vessel (Huang and Chen, 2005). This can be attributed to the limitation of air volume and gaseous exchange occurring in the culture vessel, which may affect the growth of PLBs (Kitaya et al., 2005; Saéz et al., 2012).
The smallest size of the culture vessel will have the least air capacity, and therefore, the growth of PLBs will be low as compared to that in other vessels. Only a modest amount of gas exchange occurs within the PLBs during photosynthesis due to the small volume of air in the smallest vessel. High carbon dioxide levels hinder the process of photosynthesis, which prevents the plants from synthesizing carbohydrates, thereby limiting their growth (Amoo et el., 2009). In contrast, it was observed that culture vessels with a larger size will have a higher air volume and therefore allows for free gaseous exchange. This promotes the process of photosynthesis and thus enhances plant growth in large-sized culture vessels (Dutta, Gupta and Prasad, 2010).
The relationship between the size of the culture vessels and plant growth and pigment concentration has been extrapolated in many prior studies. Claussen (2005) observed that the proline content in PLBs cultured in vessels with varying diameters reflects stress conditions. The concentration of ethylene produced by the PLBs inside the little air volume of a small-sized vessel could result in a large amount of proline. In vitro grown plantlets in a tank with restricted space have been found to produce ethylene, which slows down plantlet growth and results in tiny leaves (Islam et al., 2005; Alvarez et al., 2012). Ethylene overabundance can also inhibit the formation of PLB shoots or calli (Jackson, 2003; Xiao et al., 2011). PLBs in a small vessel showed a low growth rate. In contrast, in bigger vessels, the ethylene gas released by the PLBs can freely diffuse over a large region, resulting in a higher PLB growth. This could be because of the release of ethylene gas by PLBs, which increases proline levels (Park et al., 2002; Kozai, 2010). Larger culture vessels are capable of diluting metabolic waste accumulation and protecting in vitro grown plant tissues from stress (Islam et al., 2005; Shin et al., 2013). Carbohydrates contained in starch granules in PLBs provide energy for the plant cell to function and build the plant’s cell wall, and therefore, they are essential for PLB growth. Because starch is involved in the plant’s development in the form of ATP, PLB growth will be enhanced if the amount of starch granules is higher (Oakes et al., 2016). The authors also reported that the amount of carbohydrate content increased as the volume of culture vessels increased. In the present study, large-sized plastic and glass vessels were found to enhance chlorophyll concentration in the PLBs. Chloroplast is an important plant organelle, and chlorophyll a and b pigments are substantially required for photosynthesis. Martins et al. (2015) reported that different culture conditions affect the anatomy and physiology of in vitro growing plants. This is because of restricted gas exchange. The ideal chemical structure of the chlorophyll pigments consists of a long hydrocarbon phytol tail linked to the head (which is also called the porphyrin ring) of the chlorophyll molecule. Therefore, depending on the culture vessel conditions, the concentrations of the chlorophyll pigments are bound to change as it affects the chemical structures of the molecules in the in vitro growing cultures. It was also observed that porphyrin and carotenoid concentrations were high in cultures grown in the large-sized plastic and glass culture vessels. The photosynthetic pigments (namely, chlorophyll, porphyrin, and carotenoid) can efficiently absorb the required visible light wavelength and facilitate photosynthesis (Leyva et al., 2008). The other findings of the experiment were based on the observations of SEM. The SEM images showed that the number of stomatal pores on the PLBs was higher in the large-sized culture vessels. Gas exchange and the delivery of water and minerals in plants mainly depend on the stomata, and therefore, the number of stomata will affect the growth of the plants (Rodrigues et al., 2016). In in vitro cultures, PLBs with many stomata can absorb a large amount of CO2, which can be subsequently used in photosynthesis to generate glucose and stored as starch granules in the shoots, roots, or leaves (Leyva et al., 2008; Martins et al., 2015). The importance of large-sized vessels for culturing PLBs was also confirmed based on the observations in the small-sized culture vessel (120 ml). In the 120 ml vessel, the cultures were incapable of growing to their ideal fresh weight and showed closure the stomata. In contrast, completely grown PLBs in larger culture vessels showed fully opened stomata for gaseous exchange. The rhizoid formed on PLBs grown in glass containers (280 ml) and plastic vessels (470 ml) may aid in nutrient and water intake, both of which are necessary for the plant growth and development process.
Conclusions
Plastic culture vessels provide a reliable alternative culture vessel for the development of Dendrobium Sabin Blue orchid PLBs. The best PLB growth was attained in a 470 ml plastic jar with the highest growth index. PLBs grown in 470 ml plastic culture vessels produced the highest levels of carbohydrate and photosynthetic pigments such chlorophyll a and chlorophyll b, indicating a high photosynthesis efficiency rate. Hence, the 470 ml plastic vessel appears to be the best choice for cultivating Dendrobium Sabin Blue PLBs and produces highquality plantlets as compared to the other culture vessels used in this study.
Author contributions
Aimie Nadzirah Abd Malik designed the study, performed the experimental work, collected data, interpreted data, performed statistical analysis, and wrote the first draft of the manuscript. Sreeramanan Subramaniam supervised the work, provided technical support, and contributed to chemicals and scientific advice. Jasim Uddain, Jessica Jeyanthi James Antony, and Ankita Rajendra Parab analyzed the study, interpreted the results, and made substantial contribution to the biochemical work. Chin Chee Keong and Bee Lynn Chew edited the manuscript. All authors have reviewed and approved the final version of the manuscript.