Introduction
Several insulin activities are regulated by phosphatidylinositol 3-kinase (PI3K). Akt (protein kinase B) is a serine-threonine kinase with a pleckstrin homology domain and is an effector in some downstream signaling pathways of PI3K [1-3]. Akt also has a role in the PI3K-Akt-mTOR axis and is involved in the upregulation of the insulin-signaling pathways essential for maintaining glucose metabolism [2, 4]; it promotes glucose transport and liver glycogen synthesis, thus reducing blood glucose levels [4].
The PI3K/Akt signaling pathway plays a role in metabolism, and its imbalance is associated with developing type 2 diabetes and obesity [5]. The insulin receptor substrate (IRS)-1/PI3K/Akt signaling pathway mediates olanzapine-induced hepatic insulin resistance [6]. Increased O-linked β-N-acetylglucosamine (O-GlcNAc) is associated with insulin resistance in muscle and adipocytes [7]. Due to its characteristics, the PI3K/AKT signaling pathway is required for normal metabolism, and its imbalance leads to the development of obesity and type 2 diabetes mellitus [5]. Damage of the PI3K/AKT pathway in various body tissues leads to obesity and type 2 diabetes as the result of insulin resistance, and in turn, insulin resistance exacerbates the PI3K/AKT pathway, forming a vicious circle [8].
Increased susceptibility of the diabetic myocardium to ischemia/reperfusion-induced apoptosis/dysfunction was associated with decreased Akt activation [9]. Akt phosphorylation in islet β cells and diabetic mice promoted β-cell apoptosis [10]. Pancreatic fibroblast growth factor 21 (FGF21) protects T2DM mice by inducing PI3K/Akt signaling-dependent insulin expression and secretion [11]. Akt regulates pancreatic β-cell growth and survival, and its activation reduces the loss of functional islet mass [12].
PI3K/Akt signaling in the liver also affects FGF21-induced insulin expression and secretion [13]. Akt is associated with obesity-related target organ damage [14]. Insulin and insulin-like growth factor-1 stimulate specific arteries and are disrupted by diet-induced obesity. Following a long-term high-fat diet (HFD), insulin was a more potent activator of Akt than insulin-like growth factor-1 in the aorta, whereas, in resistance arteries, insulin-like growth factor-1 was more potent than insulin. PI3K/Akt is involved in insulin or insulin growth factor (IGF)-1-induced proliferation of intestinal crypts isolated from obese humans [15]. PI3K/Akt/Rac-1 signaling is also involved in the anti-inflammatory effect of insulin. Insulin inhibits high glucose-induced activation of p38, NF-κB, and STAT1 transcriptional activity by activating Akt-Rac-1 signaling [16].
Glycosphingolipids (GSLs) are involved in the pathogenesis of insulin resistance and associated target organ injury [17]. The GM3 ganglioside of caveolin-rich adipocytes binds to the insulin receptor (IR). Following insulin treatment, it dissociates its complex with caveolin, and lowers IR autophosphorylation [18]. The depletion of GSL in HepG2 cells affects both Akt1 phosphorylation and IR autophosphorylation by modifying the membrane microenvironment of this kinase [18].
Human hepatocyte-derived HepG2 cells are characterized by a high level of IR but a low level of caveolin. The glucosylceramide synthase inhibitors d-threo1-pheny-2-decanoylamino-3-morpholino-1-propanol (d-PDMP) and d-threo-1-(3,4,-ethylenedioxy)phenyl-2-palmitoylamino-3-pyrrolidino-1-propanol (d-EtDO-P4) lower the GM3 content, which was associated with a marked increase in Akt1 phosphorylation, and an increase in IR autophosphorylation following cell stimulation with insulin [18].
β-glucosylceramide (GC) is a GSL shown to exert immunomodulatory effects [19-27]. Oral administration of GC alleviated insulin resistance in several animal models [20, 21, 28], and preliminary results suggest a beneficial effect on type 2 diabetes and non-alcoholic fatty liver disease (NAFLD) [29].
The present study aimed to determine the effects of insulin and GC oral administration on Akt expression and metabolic syndrome characteristics in leptin-deficient ob/ob mice. Leptin regulates the long-term balance between the body’s food intake and energy expenditure [30]. Leptin-deficient ob/ob mice serve as a model of obesity-related liver steatosis and glucose intolerance [31].
Material and methods
Animals
Eight-week-old male ob/ob leptin-deficient mice, a model for obesity-associated liver steatosis and hyperglycemia [31], were purchased from Jackson Laboratories (Bar Harbor, ME). Mice were kept in the Animal Core of the Hadassah-Hebrew University Medical School. All mice were administered standard laboratory chow and water ad libitum and kept in a 12-hour light/dark cycle and at 24 degrees Celsius. The animal experiments were carried out under the supervision of the Hebrew University-Hadassah Institutional Committee for Care and Use of Laboratory Animals and with the committee’s approval.
Preparation of glycolipids
β-glucosylceramide was purchased from Avanti Polar Lipids (Alabaster, AL, USA), dissolved in a vehicle of a mixture of 30% Cremophor EL (Sigma, Rehovot,Israel) and ethanol (1 : 1) in PBS, and administered orally by gavage using a designated pipette at a dose of 2.5 mg/kg/day as described [21, 23, 25, 31].
Experimental groups
Four groups of leptin-deficient ob/ob mice (n = 6 each group) were orally administered by gavage using a designated pipette daily for four weeks: vehicle (group A); 2.5 mg/kg GC (group B); 2.5 IU regular insulin (Humulin R, Eli Lilly, group C); or a combination of GC with insulin (group D). The number of animals was calculated based on previously published data [21, 31]. Mice in all groups were followed for changes in metabolic parameters and parameters indicative of liver damage, as described [21, 23, 25]. The last treatment was administered the day before the end of the study. All studies were conducted in the morning hours.
Glucose tolerance test
The glucose tolerance test (GTT) was performed by oral administration of glucose (1.25 g/kg) at the end of the treatment period following a 6-hour fast, with repeated glucose level tests from the tail vein using a standard glucometer [31].
Liver enzymes
Serum alanine aminotransferase (ALT) was measured weekly using commercially available sticks (Reflovet Plus, Roche Diagnostic).
Triglyceride and total cholesterol levels
Triglyceride (TG) and total cholesterol serum levels were measured weekly in a fasted state using commercially available sticks with a Reflovet Plus clinical chemistry analyzer (Roche Diagnostics, GmbH, Mannheim, Germany).
Hepatic TG content
Intrahepatic TGs were quantified at the end of the study using a modification of the Folch method [32]. Quantification was followed by extracting by spectrophotometer, using a GPO-Trinder kit (Sigma, Rehovot, Israel), and levels were normalized to the protein content in the homogenate.
Serum cytokine levels
Tumor necrosis factor α serum levels were measured using a commercially available “sandwich” ELISA kit (Quantikine, R&D Systems, MN, USA) at the end of the treatment period.
Akt phosphorylation
Hepatic Akt phosphorylation was determined at the end of treatment at basal states by western blot analysis as described previously [33]. Whole tissue lysates from snap-frozen liver pieces were homogenized in modified RIPA buffer (Sigma). Lysates were cleared, and equivalent amounts of protein were used as determined by the Bradford (Bio-Rad) assay, separated by sodium dodecyl sulfate-polyacrylamide gel electrophoresis (SDS-PAGE). Proteins were detected with phospho-specific Akt (Cell Signaling, USA, #9271); β-actin (Abcam, UK, sc-47778) was used as a loading control. All membranes were developed with horseradish peroxidase-conjugated secondary antibodies (Jackson Laboratories, USA) and Western Blot-Luminol Reagent (Santa Cruz Biotechnology, USA). Western blot films were scanned and quantified using Total Lab (Phoenix, USA) software.
Results
Orally administered insulin and GC alleviated glucose intolerance and its accompanying liver injury. No significant changes in body weights were noted in all groups in the study.
Oral insulin and GC promoted hepatic Akt phosphorylation
Oral administration of GC and insulin, particularly their combination, was associated with increased protein levels and phosphorylation of Thr 308 of hepatic Akt (Fig. 1, lanes D1,2,3).
Fig. 1
Phosphorylation of Akt in the liver following administration of insulin and GC. Four groups of leptin-deficient ob/ob mice were orally administered for four weeks: vehicle (group A), 2.5 mg/kg GC (group B), 2.5 IU of regular insulin (Humulin R, Lilly, group C), and the combination of both GC and insulin (group D). Phosphorylation of Akt in the liver was determined and compared with the actin control. A synergistic effect for the combination therapy was observed (Lanes D1-D3). Proteins were detected with phospho-specific Akt (Cell Signaling, USA, #9271); β-actin (Abcam, UK, sc-47778)
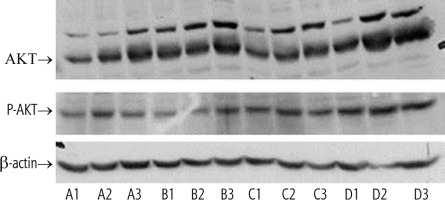
Effects of oral insulin and GC on serum levels of TNF-a
Serum TNF-α levels varied from 242 pg/ml in group A to 264, 200, and 215 pg/ml in groups B, C, and D, respectively (p = NS, for B vs. A; and p < 0.05, for C and D vs. A) (Fig. 2).
Oral insulin and GC alleviated glucose intolerance and hyperlipidemia
Oral administration of insulin and GC, particularly in combination, significantly reduced the areas under the curve (AUC) of the GTT. AUC was 45649 in group A vs. 25759, 24916, and 19950 in groups B, C, and D, respectively (p ≤ 0.05 for B, C, and D vs. A) (Fig. 3A). GTT was reduced in mice that received the combination of GC and insulin compared to mice that received either GC or insulin (p < 0.01, for D vs. B and C).
Fig. 3
Effects of oral administration of insulin and GC on glucose tolerance and hyperlipidemia. Mice in all experimental groups underwent a glucose tolerance test (GGT), and data are shown for areas under the curve. Following both oral insulin and oral GC, GGT decreased (A). A synergistic effect of the combination therapy with insulin and GC was observed (p ≤ 0.05 for B, C, and D vs. A; p < 0.01 for D vs. B and C). Both oral insulin and oral GC were associated with decreases in serum triglyceride (TG) levels (B, p < 0.05, for B vs. A) and with reductions in serum total cholesterol (C, p < 0.05, for B vs. A; and p < 0.005, for C and D vs. A). The changes were more significant in insulin-treated mice
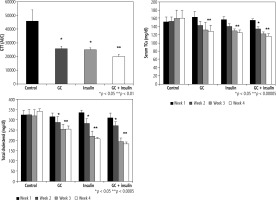
Serum TG was significantly lower in all treated groups than in vehicle-treated mice (Fig. 3B, 129, 125, and 116 mg/dl in groups B, C, and D, respectively, compared to 160 mg/dl in group A, p < 0.00005).
Four weeks after initiation of the trial, total serum cholesterol levels were 341 mg/dl in group A vs. 254, 207, and 183 mg/dl in groups B, C, and D, respectively;differences were more profound in insulin-treated mice (Fig. 3C, p < 0.05, for B vs. A; and p < 0.005, for C and D vs. A).
Effects of oral insulin and GC on ALT levels
As early as week 2 of the study, ALT levels were lower in groups B, C, and D (p < 0.01) than in group A. After four weeks of treatment, more significant differences were observed between the groups (420 in group A vs. 215,188, and 168 u/l in groups B, C, and D, respectively; differences were more significant in insulin-treated mice (p < 0.005, for B vs. A; and p < 0.001, for C and D vs. A). The hepatic TG content was reduced by oral insulin and by the combination of insulin and GC, but not by GC alone, 10.4% in group A vs. 10.5%, 5.8%, and 7.7% TG in groups B, C, and D, respectively (Fig. 4B, p = NS, for B vs. A; and p < 0.05, for C and D vs. A).
Fig. 4
A) Changes in ALT serum levels following administration of insulin and GC. ALT serum levels were measured in all experimental groups. Decreases in insulin-treated and GC-treated mice were observed as early as the second week of therapy. At the end of four weeks of treatment, further reductions were observed, which were more significant in insulin-treated mice (p < 0.005, for B vs. A; and p < 0.001, for C and D vs. A). The hepatic triglyceride (TG) content was measured in all mice at the end of the four-week treatment period (B). A significant reduction in liver TGs was noted for insulin-treated mice (p = NS, for B vs. A; and p < 0.05, for C and D vs. A)
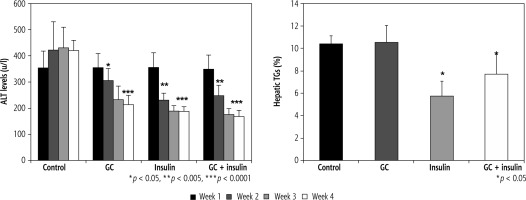
Discussion
Oral administration of insulin or GC, and the combination of both, increased Akt expression in the liver and were associated with a trend for alleviating glucose intolerance and ameliorating liver damage in an ob/ob leptin-deficient mouse model of the metabolic syndrome and NAFLD.
The present study results further support the importance of the Akt pathway in alleviating glucose intolerance and the associated hyperlipidemia and liver damage. PI3K/Akt signaling is relevant in skeletal muscle, adipose tissue, the liver, the brain, and the pancreas, suggesting that it can serve as a therapeutic target for obesity and type 2 diabetes mellitus [5].
Alterations in the PI3K/Akt pathway, found in damaged tissues, are linked to obesity, insulin resistance, and type 2 diabetes. Insulin resistance can further impair the PI3K/Akt pathway, thus forming a vicious circle [5]. Anti-diabetic compounds that target hepatic insulin resistance in type 2 diabetes are associated with activated hepatic AMP-activated protein kinase and PI3K/Akt pathways [34]. The anti-diabetic effects of other compounds were associated with the activation of Akt signaling [35].
The combination therapy of GC and insulin showed a synergistic effect for promoting Akt expression in the liver and alleviating glucose intolerance. An adjuvant effect of GC was previously described in the ob/ob model when co-administered with anti-CD3 [31] and with other drugs and vaccines [25, 36]. These effects were attributed to the potential immunomodulatory effect of GSLs [19, 37]. They also support a potential oral-immunotherapy-based mechanism of action recently described for several compounds [21, 25, 29, 37-48].
The use of GSLs as potential activators or inhibitors of Akt may be related to their structure. Mimics of phosphatidyl were synthesized as Akt inhibitors based on a beta-glucoside scaffold. These compounds possessed one or two lipophilic moieties of different lengths at the anomeric position of glucose and an acidic or basic group at C-6 [49].
Similar to previously published data of studies in both animals and humans [19-23, 25-29, 33, 50-59], in the present study, GC exerted a beneficial effect on liver enzymes and metabolic parameters. However, the effect on liver steatosis observed herein was not demonstrated. This discrepancy may be due, at least in part, to differences in environments [50]. Different effects on liver enzymes and steatosis per se may result from different underlying mechanisms of the pathogenesis involved. The findings also corroborate the notion that most patients with steatosis do not develop non-alcoholic steatohepatitis (NASH) and the observation that NASH also occurs in lean patients [60, 61]. Whether the beta glucosylceramide used in the study has any unique structural feature that might make it an activator of Akt is yet to be determined [17, 19-23, 25-27, 52, 62-68].
Orally delivered insulin mimics the natural route of endogenous insulin secreted by the pancreas into the portal vein and directly to the liver [69]. Insulin therapy is central to diabetes management; however, no commercially available oral formulation is available [70].While attempts are being carried out to achieve clinically meaningful results with oral formulations, the present results support the concept that oral insulin exerts a beneficial effect on liver steatosis and liver damage in NAFLD. The data support potent adjuvants that may synergize their effects by working via similar mechanisms, such as promoting Akt phosphorylation and synergistic pathways.
Treatment with insulin was associated with an anti-inflammatory shift suggesting an orally-immunomodulatory mechanism [37, 46]. The beneficial effects of insulin were associated with a reduction in TNF-α serum levels. TNF-α contributes to the pathogenesis of glucose intolerance associated with obesity [41, 71]. HFD induces glucose intolerance and is associated with increased plasma levels of TNF-α and reduced Akt signaling [72]. The alleviation of insulin resistance in adipocytes was associated with glucose uptake, and increased insulin sensitivity in TNF-α-treated adipocytes was associated with expression of the miR-721-PPAR-gamma-PI3K/AKT-GLUT4 signaling pathway [73]. Inhibition of Akt phosphorylation demonstrated effectiveness in ameliorating glucose intolerance caused by TNF-α [71]. TNF-α induced glucose intolerance via inhibition of Akt/eNOS/NO signaling and was suggested as a target for alleviating glucose intolerance and vascular complications associated with obesity-related conditions [72]. The data suggest that further studies are required to delineate the correlation between TNF-α and Akt pathways.
The study did not include an insulin tolerance test (ITT), which can shed further light on the potential effect of the treatment on glucose intolerance. Future studies will include broadened cytokines, cellular, and signaling molecule profiles, as well as pathological studies, which are expected to dissect better the mechanisms underlying the findings. Comparing the effect of basal versus insulin-stimulated states on Akt expression is expected to further explain its role in the pathogenesis of glucose intolerance. Densitometry studies can improve the accuracy of the reported findings and are subject to future studies.
In summary, the present study results establish Akt expression in the liver as a therapeutic target for treating glucose intolerance, hyperlipidemia, and associated liver damage. Oral administration of insulin with GSLs significantly promoted phosphorylation of Akt, alleviating glucose intolerance and NAFLD in an ob/ob leptin-deficient mouse model of the metabolic syndrome and NAFLD. As preliminary data with oral delivery of insulin [74] and oral GC [29] showed promising results in type 2 diabetes and NASH, their combination may provide a potential therapy to be evaluated in further clinical trials.