Introduction
Overweight and obesity are serious illnesses that result from an imbalance between energy intake and expenditure, leading to the subsequent accumulation of excess fat in the body [1]. The prevalence of overweight and obesity have been increasing worldwide at an alarming rate over the past few years and have become major public health concerns among all global populations and age groups [2]. Obesity is associated with several diseases, including coronary heart disease, type 2 diabetes, metabolic syndrome, stroke, and cancers, and it is therefore also associated with significantly increased morbidity and mortality rates [3].
Lifestyle changes and pharmacotherapy interventions have been investigated as potential obesity treatment methods [4]. However, despite some short-term benefits, drug treatments have been associated with various problems, including weight gain after drug discontinuation, intolerable side effects, and addiction [5]. Due to the complex pathogenesis of obesity, lifestyle modification interventions have also had disappointing results, and there remains a need for simple, acceptable obesity treatments [5].
Herbal supplements [6], natural products, and medicinal plants, including both crude extracts and compounds isolated from plants, are the most common complementary and alternative methods used to induce weight loss and prevent dietary obesity, and they are widely used across the globe. Plants contain several components with different anti-obesity and antioxidant properties that can affect body metabolism and fat oxidation. Furthermore, plant extracts have been widely used in recent years to better manage obesity [7, 8].
The stinging nettle plant, Urtica dioica (UD), is a species of the family Urticaceae, whose roots, leaves, and seeds are used in folk treatments for many diseases [9]. Steroids [10], terpenoids [11], phenylpropanoids, coumarins [12], and polysaccharides [13] have been isolated from their roots, and 7 flavanol glycosides (kaempherol-3-O-glucoside and -3-O-rutinoside; quercetin-3-Olucoside and -3-O-rutinoside; and isorhamnetin-3-O-glucoside, -3-O-rutinoside, and -3-Oneohesperidoside) [10] have been isolated from their flowers. These major flavonoid glycosides have immunostimulatory, anticarcinogenic, anti-inflammatory, antioxidant, and antiallergenic properties [14, 15]. UD has been demonstrated to have anti-inflammatory [16], acute diuretic, natriuretic, and hypotensive effects [17], and it has also been reported to treat stomach pain [18], liver failure [19], rheumatic pain, colds, and coughs [20].
Aim
The present study sought to investigate the preventive effects of UD seed extract on high-fat diet-induced (HFD) obesity in rats.
Material and methods
Animals
Thirty-two 4–5-week-old male Wistar albino rats were provided by the animal house of the Medical School of Yuzuncu Yil University. Animals were housed at 20 ±2°C in a 24-h light/dark cycle. All animals were kept in stainless cages and fed with a wheat-soybean-meal-based diet. Water and feed were offered ad libitum. The animals received humane care according to the criteria outlined in the ‘Guide for the Care and Use of Laboratory Animals’ prepared by the National Academy of Science and published by the National Institutes of Health. Ethical regulations were followed in accordance with national and institutional guidelines for the protection of animal welfare during experiments. Ethical approval was obtained from the Animal Experiments Local Ethics Committee of Van Yuzuncu Yil University (26.01.2017/01).
Plant material
For extraction, the UD seeds were ground in an electric mill. UD seed extract was obtained with ether [15]. UD extract was given by daily orogastric gavage to 300 mg/kg body weight [21, 22].
High-fat diet
A standard pellet feed obtained from a special commercial feed manufacturer was milled. Subsequently, 300 g/kg of butter was added to the feed to form a high-calorie (approximately 4000 kcal/kg), high-fat (60% of its energy consisted of saturated fat) pellet feed that was fed to all of the rats in the 2 HFD groups [23].
Experiment design
Male Wistar albino rats (n = 32) were randomly divided into 4 groups of 8 rats each.
Control group: During the experiment, the rats received a standard pellet diet and tap water ad libitum.
HFD group: During the experiment, the rats received the high-fat pellet diet ad libitum.
HFD + UD group: During the experiment, the rats received UD extracts (300 mg/kg bw) and the HFD.
UD group: During the experiment, the rats received UD extracts (300 mg/kg bw) and standard pellet diet.
UD extracts were given by daily orogastric gavage. After 75 days, all the rats were weighed, euthanised, and necropsied, and then tissue and blood samples were taken.
Biochemical examination
For biochemical analysis, blood and tissue samples were taken from the rats at the end of the experiment. Alanine transaminase (ALT), aspartic transaminase (AST), alkaline phosphatase (ALP), lactate dehydrogenase (LDH), γ-glutamyl transferase (GGT), cholesterol, triglyceride, high-density lipoprotein (HDL) cholesterol, and high-density lipoprotein (LDL) cholesterol activities were examined.
Histopathological examination
Tissue samples taken from sacrificed rats were fixed in 10% neutral formalin and embedded in paraffin by routine methods. Sections 5 µm in thickness were cut for histological examination. The slides were examined and photographed using an E-400 light microscope (Nikon, Japan) equipped with a DS-Ri2 video camera and DSU3 microscope video camera controller (Nikon, Japan). Each section was evaluated for histopathological findings such as hepatic steatosis, necrosis, inflammation, and fibrosis. Ten 200× light microscopic fields were viewed on each section and scored for the severity of hepatic steatosis, according to the following criteria [24] for hepatic steatosis: grade 0, no fat; grade 1, steatosis occupying less than 33% of the hepatic parenchyma; grade 2, 34–66% of the hepatic parenchyma; and grade 3, more than 66% of the hepatic parenchyma.
Oil Red O staining
The GBL (Gül Biology Laboratory) commercial kit was used. Sections were stained according to the procedure specified in the kit.
Statistical analysis
All data were expressed as mean ± standard deviation (SD). The statistical analyses were made using SPSS 20.0 for MS Windows. One-way ANOVA statistical test was used to determine the differences between means of the treatment and the control group, accepting the significance level at p ≤ 0.05.
Results
Histopathological findings
The histopathological examinations showed a normal histological structure in the liver tissues of the rats in the control group (Figure 2 A). However, the rats in the HFD group all had sharply circumscribed vacuoles of different sizes in their hepatocyte cytoplasm, and many also had coagulative necrosis in their liver parenchyma. The nuclei of the necrotic hepatocytes were darkly stained and pyknotic, and their cytoplasms were eosinophilic. Hydropic degeneration was also present, to varying degrees, in hepatocytes of the rats in the HFD group. Some of the rats in the HFD group also had congestion in some of their vena centralis, as well as dilatation in their sinusoids (Figure 2 B). All the rats in the HFD + UD group had similar histopathology findings to the rats in the HFD group, but to a significantly lesser extent. In particular, their fat vacuoles were considerably reduced and were restricted to the hepatocytes in the centrilobular region. Similarly, there was a noteworthy reduction in the amount of necrosis (Figure 2 C). The histopathological examinations showed a normal histological structure in the liver tissues of the rats in the control and UD groups (Figure 2 D).
Figure 2
A – Normal histological structure of the liver in the Control group. B – Sharply circumscribed fat vacuoles of varying sizes (arrows) in the hepatocytes, and coagulative necrosis (arrowheads) and vena centralis congestion in the HFD group. C – Sharply circumscribed fat vacuoles (arrowheads) of varying sizes observed in the hepatocytes of the centrilobular region in the HFD + UD group. D – Normal histological structure of the liver (H&E) in the UD group
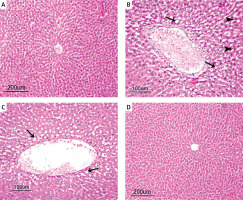
Because severe hepatic steatosis was observed in both groups of rats that were fed the HFD, we aimed to clarify whether the fat accumulation was induced in the liver via staining of the liver tissues with Oil Red O. This clearly demonstrated that there were Grade 2 triglyceride deposits in the hepatocytes of the rats in the HFD group and Grade 1 triglyceride deposits in the rats in the HFD + UD group. No deposits were detected in the rats in the control or UD groups (Figures 3 A–D).
Biochemical findings
Serum AST, ALT, ALP, and LDH levels are biomarkers of liver damage that can function as biochemical indicators of liver-protective effects. Serum ALT and ALP levels were significantly higher in the HFD group than in the other 3 groups. Although they were substantially lower in the HFD + UD group, they were still higher than in the control group. There were no statistically significant differences between the serum AST and LDH levels between any of the groups. The mean values of the control and intervention groups with the standard errors are shown in Table I.
Table I
Effect of HFD and UD extract on serum AST, ALT, LDH, and ALP levels
The triglyceride, high-density lipoprotein (HDL), low-density lipoprotein (LDL), and cholesterol levels of the rats in all 4 of the groups are shown in Figures 4–7. When compared to the rats in the control group, rats of the HFD group had higher levels of triglyceride, LDL, and cholesterol, while HDL levels were reduced. However, the triglyceride and LDL levels were lower, and the HDL and cholesterol levels were higher, in the rats in the HFD + UD group when compared with those in the HFD group.
Figure 4
Effects of the HFD and UD on the triglyceride levels. Each value represents the mean ± SD. Different superscripts in each column represent statistical significance at p < 0.05
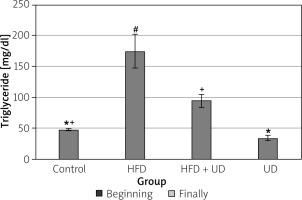
Figure 5
Effects of the HFD and UD on the HDL levels. Each value represents the mean ± SD. Different superscripts in each column represent statistical significance at p < 0.05
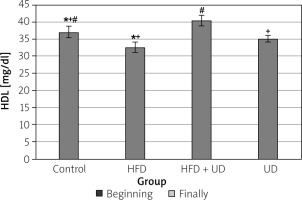
Discussion
Malnutrition due to increased consumption of ‘ready-to-eat’ foods containing high levels of fat and carbohydrates can cause hepatic steatosis and obesity [25], and human studies have shown that an HFD, defined as one in which more than 30% of dietary energy comes from fats, can induce obesity [26]. Several different methods have been investigated to reduce body weight and its associated complications. Disappointing results from both lifestyle modifications and pharmacotherapy treatments have forced researchers and physicians to look for new, safe, and effective treatment alternatives [5]. Many plants have, therefore, been examined for anti-obesity properties, along with potential mechanisms to isolate and extract their pure components. Herbal remedies stand out as a potentially effective option to reduce body weight and body fat [27]. The present study, therefore, sought to investigate the histopathological and biochemical preventive effects of UD extract on HFD-induced obesity in rats.
Plants and their mineral or chemical components and extracts, especially those commonly used in food, are widely used in alternative and complementary medicine to prevent or treat obesity and its related complications [28]. For instance, human studies have reported significant weight loss associated with Nigella sativa [29], extra virgin olive oil [30], and pistachio nut [31] treatments. Studies on rats [32–34] and mice [2, 35] have also reported considerable weight loss in association with plants and plant extracts. The anti-obesity mechanisms of plants include reduced lipid absorption and energy intake, increased energy expenditure, decreased pre-adipocyte differentiation and proliferation, decreased lipogenesis, and increased lipolysis [36]. Virgin olive oil [30], capsinoids [37], and Lycium barbarum [38] lead to higher fat oxidation in humans, while a number of polyphenols obtained from tea extracts (e.g. L-epicatechin, epicatechin-3-gallate, epigallocatechin, and epigallocatechin-3-gallate) have demonstrated strong inhibitory effects against pancreatic lipase, thereby leading to weight loss [39–41].
It was previously demonstrated that rats that consumed a HFD gained weight faster than those that consumed a low-fat diet [42, 43]. In the present study, the rats were fed a HFD that consisted of 60% fat for 10 weeks. After 8 weeks of consuming an obesity-inducing diet, the rats that showed a body weight increase of at least 20% when compared to the control group were regarded as obese [44, 45]. The present study found that the rats fed a HFD weighed 40% more than the rats in the control group. This finding was consistent with other research. For example, one study found that mice that were fed a HFD diet for 8 weeks weighed approximately 50% more than those in the control group, which were fed a normal diet [46], while another study reported that weight gain was significantly higher in rats fed a HFD for 4 weeks than in the rats in the control group [47]. Taken together, these results indicated that increases in body weight may be related to HFDs [48, 49].
Obesity treatments are considered successful if they prevent further weight gain, reduce initial weight by 5–10%, and provide sustained long-term weight loss [2]. However, the efficiency of new obesity treatments should first be evaluated by their effects on body weight [50]. In the present study, weight gain was suppressed by 26% in the rats in the UD + HFD group when compared to the rats in the HFD group. It can therefore be concluded that the UD extract was successful in treating obesity because it prevented further weight gain.
UD can control obesity and its complications [51]. Aqueous ethanolic extracts of UD are demonstrated to be effective for weight reduction in those with obesity by reducing the pre-inflammatory factor of chemerin and obesity-related factors [52]. UD protects against HFD-induced obesity through mechanisms involving lipid accumulation and glucose metabolism in skeletal muscle, liver, and adipose tissue [53]. Madadi Jaberi et al. reported that hydro-alcoholic extract of UD significantly reduces the levels of apelin and high sensitivity C-reactive protein, which points to its effective role in controlling body fat percentage and obesity [54]. UD plant attenuates HFD-induced weight gain through its effects on gut microbiota composition and diversity. A high proportion of soluble and insoluble fibre and phytochemicals in UD may serve as substrates for gut bacteria, resulting in proliferation of beneficial bacterial taxa or metabolites that affect host cellular mechanisms [55]. Based on results from the present study, it is believed that the reduction in body weight as a result of UD treatment is due to the above-mentioned mechanisms.
Hepatic steatosis is a well-known pathology in severely obese human patients, and it is particularly associated with visceral adiposity and diabetes [56]. Dietary fat intake is probably responsible for increased adipocytes [43]. Simple steatosis, steatohepatitis, severe fibrosis, and cirrhosis are typically observed clinicopathologically in patients with fatty liver disease [57], which is diagnosed via histopathological examination and is defined as the presence of fatty vacuoles in more than 5% of hepatocytes [58].
Hepatic steatosis has also been reported in rats [56]. For instance, Xu et al. [24] reported that Grade 1 hepatic steatosis (defined as less than 33% steatosis of the hepatic parenchyma) occurred in the fourth week of a HFD and Grade 2 hepatic steatosis (i.e. more than 33% steatosis of the hepatic parenchyma) developed in the eighth week. Moreover, hepatic steatosis continued to gradually increase from the 12th week to the 48th week. In addition, mononuclear cell infiltrations, vascular dilatation [56], and congestion [59, 60] have also been reported in rats fed a HFD. The results of the present study were consistent with these previous findings.
Recent research has reported that some plants have prevented hepatic steatosis in an experimentally generated fatty liver model with a HFD [61]. Consistent with this, in the present study, significantly more hepatic steatosis was observed among rats in the HFD group than among the rats in the HFD + UD group.
Studies have also found that the immunomodulatory and antioxidant effects of the UD extract provide some protection via immunomodulatory and antioxidant effects against coagulative necrosis, lipid peroxidation, and the liver degeneration and fibrosis associated with carbon tetrachloride toxicity. In addition, UD extract causes a significant reduction in the number of SMA-positive perisinusoidal cells with myofibroblastic transformation and lysosomal enzyme activity suggesting fibrogenesis [62]. Yener et al. [9] found that UD extract significantly improved the hydropic degeneration, necrosis, fat degeneration, bile duct proliferation, and periportal fibrosis caused by aflatoxicosis through a decrease in MDA, enhancement in the activities of CAT, GR, and SOD, decreases in the levels and activities of serum AST, ALT, and GGT, and prevention of the formation of ROS or scavenges. In this study, similar findings were decreased as a result of the UD extract treatment.
Increased hepatic triglyceride levels have been reported to cause hepatic steatosis in some obese individuals [63]. Natural products, such as pistachio nuts [31], psyllium fibre [64], black Chinese tea [39], Camellia sinensis [65], yacon syrup [66], olive oil [30], and fenugreek seed [67], have all been shown to reduce triglyceride levels. An anti-hyperlipidaemic effect of UD extract has been demonstrated by many studies in rats [68–71]. The presence of antioxidants in UD extract components may play a role in the hypolipidaemic effect. It has been stated that this hypothesis can be considered as one of the mechanisms by which this extract can regulate lipid homeostasis [51, 72]. Consistent with these findings, the present study saw significantly decreased triglyceride levels in the rats treated with UD extracts, suggesting that UD extracts cause histopathological changes in the liver.
Previous research has shown that the serum levels of AST and ALT were significantly higher in obese rats than in rats with normal body weight [48]. These increased levels, as well as the increased LDH activity, can be attributed to the changing structure of liver cells, which are intracellular enzymes that are present in only a small amount of serum [73]. This is because when liver cells are damaged by autolytic destruction or cellular necrosis they leak into the plasma [74]. However, various doses of methanolic UD extracts have been reported to reduce serum ALT, AST, and LDH in mice with cisplatin-induced toxicity [73]. Similarly, another study found that UD extracts significantly reduced serum AST and ALT values in hypercholesterolaemic mice, which was attributed to the antihepatotoxic potential of the UD extract [75]. UD extract has no apparent negative effects on liver enzymes and has also been shown to decrease enzyme levels in rats fed a HFD [76]. Consistent with these results, the present study found that serum ALT and ALP levels were highest in the HFD group and second highest in the HFD + UD group. However, in contrast to previous studies, no significant difference was found in the serum AST and LDH levels in any of the groups. It is possible that the duration of the study may have affected the serum ALT level findings. As a matter of fact, one study observed significantly increased serum ALT levels in rats fed a HFD in the 4th, 12th, and 16th weeks of the study, but no significant changes were observed in the 8th, 36th, or 48th weeks [24].
A HFD causes dyslipidaemic changes by increasing the levels of serum triglycerides, total cholesterol, and LDL cholesterol and lowering the serum HDL levels. Dyslipidaemic changes in obesity may be due to the increased entry of unesterified fatty acids into the liver caused by increased triglycerides [77]. It has been shown that serum triglyceride, total cholesterol, LDL cholesterol, and very-LDL cholesterol levels increase, while HDL cholesterol levels decrease in rats fed an HFD [78]. Similarly, Amin and Nagy [48] reported that serum triglyceride, total cholesterol, and LDL cholesterol levels increased, and HDL cholesterol levels decreased in rats fed an HFD. Consistent with these findings, the present study observed that the serum LDL, cholesterol, and triglyceride levels were significantly higher, and the HDL cholesterol level was significantly lower in the rats in the HFD group than in the control group, while in the HFD + UD group, the serum LDL and triglyceride levels were lower and HDL levels were higher than in the HFD group.
Serum cholesterol levels were higher in the HFD + UD group than in the HFD group, suggesting that the cholesterol levels increased as a result of the UD extract treatment. Although the cause of these unwanted results is unknown, a connection between the type of rats, the presence of diabetes, the method of extract preparation, and the duration of the study has been hypothesised [59]. In a study conducted in fish, different doses of UD given for 4 weeks did not cause a significant change in triglyceride and cholesterol levels. However, when the same doses were applied for 8 weeks, a decrease was observed in triglyceride levels, but no change was detected in cholesterol levels. The difference in serum cholesterol was attributed to the presence of plant sterols such as stigmasterol, campesterol, and phenolic compounds [79]. It has been stated that the reduction of triglycerides may be due to carvacrol in nettle, which can have a greater effect on lipogenesis than cholesterol biosynthesis [80]. As a result, the cholesterol level is likely to vary based on numerous parameters.
Conclusions
Efforts to combat obesity have typically been disappointing. Complex pathogenesis, misdiagnosis, mistreatment of the disease, and several unwanted effects associated with various treatment methods suggest that obesity cannot be widely treated with simple drug therapy. Moreover, approaches tailored to the needs of individual patients, and changes in eating habits and energy are typically necessary to help overweight or obese patients lose weight. In the present study, UD extract partially prevented obesity and its associated effects. UD extract decreased weight gain, decreased hepatic steatosis, and significantly improved serum triglyceride, HDL, and LDL levels in rats fed an HFD. However, the UD extract also increased the cholesterol levels of the rats; hence, by itself, it is presumably an insufficient treatment for obesity due to the complex and multifactorial nature of the disease.