INTRODUCTION
The relationship between neuroanatomy of speech processing and handedness has been a focal point of clinical neuroscience research [1-3]. Broca [4] initially argued the concept of hemispheric dominance in speech production while Mesulam [5] identified the inferior frontal gyrus bilaterally as the key anatomical source of speech. Even though previous studies have confirmed the lateralization of speech, particularly within the left hemisphere [6, 7], the novel models of neural correlates of language processing clearly demonstrate that both hemispheres contribute to various aspects of language processing. These models also highlight important computational differences between the left- and right-hemisphere systems [8].
Following Broca’s basic premise and the characterization of left-hemispheric language regions in right-handed individuals [4], speech hemispheric lateralization is widely regarded as being associated with handedness [9]. The dominance of left hemisphere in various aspects of speech production and perception exemplifies the functional hemispheric asymmetries present in the human brain [1]. Traditional theories of language lateralization suggest that it shares ontogenetic determinants with handedness due to their correlated nature [2]. However, the extent of this correlation varies depending on the assessment methods employed for each trait, and the neurological mechanisms underlying language lateralization differ from those associated with handedness [1]. While there is some overlap in genetic influences, both traits also exhibit significant independent developmental influences [3].
Research involving substantial participant cohorts has identified a continuum of language lateralization patterns, ranging from strongly left-dominant to strongly right-dominant [10-14]. Estimates of variability in hemispheric language dominance across handedness categories indicate that approximately 95% of right-handed individuals exhibit left-hemisphere dominance, compared to approximately 76% of left-handed individuals [10, 13, 15-17]. Research conducted by Knecht et al. [10, 16] explores the natural distribution of language dominance across both hemispheres in right- and left-handed individuals. Their findings revealed that nonstandard right-hemispheric language dominance increases linearly with the degree of left-handedness, starting from 4% among strongly right-handed individuals, rising to 15% in ambidextrous individuals, and peaking at 27% in strongly left-handed individuals [10, 16]. These results underscore the relationship between handedness and language dominance, while also highlighting that a significant proportion (73%) of strongly left-handed individuals still display typical left-hemispheric language dominance, akin to most right-handed individuals. This research was pivotal in challenging the notion that language processing is inherently relegated to the left hemisphere, as evidenced by the presence of right-hemisphere dominant speech networks in some individuals. Furthermore, it suggested that hand dominance and speech lateralization may represent distinct biases, with lateralization profiles not solely determined by one another [3].
However, the study of Somers et al. [18], did not support the hypothesis that handedness is related to direction of hemispheric speech dominance. Their findings revealed that the degree of hand preference does not reliably predict the extent of language lateralization. Nonetheless, they observed that greater left-handedness correlated with increased variability in cerebral dominance directionality [18].
Historically, invasive methods, such as direct cortical stimulation [19], chronically placed subdural electrodes [20] and Wada test [21] were used to define the dominant hemisphere in neurological patients. Currently, advances in imaging techniques – such as functional magnetic resonance imaging (fMRI), functional transcranial Doppler sonography, and positron emission tomography (PET) – have made larger studies on the neuroanatomy of speech processing more feasible [22]. However, transcranial magnetic stimulation (TMS) is the only tool that allows for detecting both neural excitation and neural inhibition, capturing subtle metabolic processes [23].
In this study, we chose repetitive TMS (rTMS) to investigate the involvement of the left and right hemispheres in speech production. We previously detailed a step-by-step algorithm outlining a simplified methodology for inducing speech arrest using rTMS [24]. This safe and user-friendly tool operates based on the principle of magnetic field induction [25]. As the coil is placed over a person’s head, a pulse of current passes through the coil, generating an impulse that penetrates the scalp and reaches the brain, inducing the secondary ionic current that causes neurons in the targeted area to depolarize [26]. TMS offers a distinct advantage by allowing the facilitation or inhibition of cortical activity [23] which other research tools, such as fMRI and PET, lack. In our study, rTMS effectively induced speech arrest in participants, enabling an evaluation of the left and right hemispheres’ involvement in speech production. This method seems to be the most appropriate for our research, as patients remain conscious throughout the procedure, allowing for real-time examination. Understanding brain function in healthy individuals facilitates further studies and may lead to new therapeutic approaches to the treatment of impaired linguistic functions, particularly given successful rTMS treatment methods in psychiatry [27].
The difference between speech and language should be clearly stated, as these two aspects of brain functioning are separate [28]. Language encompasses the understanding and expression of words and sentences to communicate thoughts and information [29]. It manifests in various forms, including spoken, written, or sign language. Speech, on the other hand, specifically pertains to the creation of vocal sounds, involving both motor (articulatory) and linguistic abilities [29]. It is possible to experience difficulties with speech while retaining intact language skills, as seen in individuals with physical impairments affecting articulation who can still convey thoughts through written communication.
Different domains of language include phonology, morphology, lexicology, semantics, syntax, and pragmatics while the domains of speech consist of voice, articulation, resonance, fluency, and perception. In our study we specifically examine speech processing [29].
Handedness is one of the best-known and most studied uniquely human asymmetries [30]. Approximately 90% of the population is right-handed and 10% is left-handed [31]. Handedness can be self-reported [32] or assessed by the Stanley Coren Handedness Inventory [33, 34], a 14-item tool designed for this purpose [35, 36]. Additionally, scientific assessments of handedness may involve recordings of lateral asymmetries in the surface electromyogram (EMG) during isometric contractions [37] or evaluations of H-reflex in the dominant upper limb [38]. However, the Edinburgh Handedness Inventory (EHI) [39] is most widely used tool and when administered carefully [40], it allows for comparability of results.
Efforts to identify factors influencing cerebral lateralization and handedness have yielded varied outcomes. A recent meta-analysis of genome-wide association studies on handedness [41] estimated that the number of genetic loci influencing handedness is at least 40, potentially reaching up to 100. These findings challenge theories that propose single-gene explanations for handedness [42]. Instead, handedness likely signifies an imbalance in cortical processing and neurological organization, rather than a morphological asymmetry of the hands themselves [43].
There is limited literature available on research involving left- and mix-handed individuals. Existing studies, which used intracarotid amobarbital procedure, fMRI and transcranial Doppler sonography, have indicated with statistical significance that in left-handed people, the neuroanatomy of speech processing is atypical [10, 44, 45].
The involvement of the left and right hemispheres in speech production and its correlation with handedness remains ambiguous in healthy individuals [18]. Previous studies have primarily focused on neurological patients and have produced conflicting results. Repetitive transcranial magnetic stimulation (rTMS) has long been used in research on speech arrest [36, 46-50]. Notably, only one study by Khedr et al. [34], used rTMS to evaluate the correlation between neuroanatomy of speech processing and handedness; however, this work lacked a detailed investigation of the results and further analysis.
Additionally sex may play a potential role in the lateralization of verbal functions [51, 52]. Preliminary analyses suggest that females are more prone to exhibit symmetrical patterns of connections, while males are more prone to hemispheric language dominance [52].
In our study, we aimed to induce speech arrest using a specific of rTMS protocol that we previously refined [24]. We intended to determine the effects of stimulation, examining the correlation between handedness and its exact laterality index and investigating the contribution of the left and right hemispheres to speech production in healthy individuals.
MATERIAL AND METHODS
Study group
Fifty-eight healthy volunteers participated in our study, comprising 30 males and 28 females. All participants were Caucasian Polish native speakers and medical students with a median age of 22 years (range 18-34 years). Volunteers completed a screening questionnaire [53] with its subsequent update [54]. Participants who indicated any contradictions for undergoing the TSM procedure were excluded from the study. The bioethics committee of Poznan University of Medical Sciences approved the study, which was conducted in accordance with the Declaration of Helsinki. The participants provided their written informed consent.
Study design
Handedness and motor thresholds of the volunteers was determined according to previously established protocols [24]. Subsequently, we analyzed the involvement of the left and right hemispheres in speech production through rTMS. This original project [24] was developed by implementing the protocols of Epstein et al. [32] and Pascual-Leone’s et al. [55], along with the original improvements based on the latest findings from both navigated transcranial magnetic stimulation (nTMS) [56] and rTMS studies related to speech production.
Determination of handedness
Following neurophysiological examinations, volunteers completed Oldfield’s EHI [39]. Based on the obtained Laterality Index (LI), handedness deciles were assigned to each participant. Participants qualified as right-handed if their LI ranged from 48 to 100 (1st right decile to 10th right decile) and as left-handed with LI from –100 to –28 (10th left decile to 1st left decile) and as mix-handed when LI ranged from –28 to 48 (middle decile). Participant characteristics are presented in Table 1.
Table 1
Participant’s characteristics due to handedness*
Determination of stimulation threshold
The active motor threshold (MT) is defined as the stimulator output necessary to elicit at least five consecutive visible twitches of any hand muscle on the contralateral side to the stimulation, with a single pulse applied over the motor cortex. According to Epstein MT estimates obtained using active and resting motor potential do not differ by more than 2% of the maximum stimulator output [32, 54, 57]. The intensity of the subsequent rTMS stimulation was based on the individual’s MT.
Speech arrest
We adhered to heuristic criteria for determining stimulation placement [32, 49]. A MagPro R30 magnetic stimulator (Medtronic) was employed. The stimulation site was located 8 cm above the tragus, tangential to a preauricular line [55]. We used a round coil (C-100) with a diameter of 110 mm, which produced a biphasic pulse. The maximum generated magnetic field reached 4 Tesla during the steep rise of the pulse near the coil surface and within approximately 1 cm of the central axis.
As participants counted downwards from 20 to 10, the stimulation was triggered randomly over the left hemisphere followed by stimulation over the right hemisphere after a 15-minute break. The stimulation was delivered at a frequency of 2 Hz and lasted 2 seconds. The protocol was standardized for all participants. We determined the strength of the stimulation as 120% of each individual’s MT. If the initial stimulation was unsuccessful, meaning that no effect on speech was observed, a second attempt was made with 150% of MT. The procedure was video-recorded as recommended [58].
Evaluation of the results
Three independent investigators as well as participants assessed the video recordings. If positive stimulation was observed, the investigators further classified its type. ‘Full speech arrest’ was declared if a subject failed to produce at least one numeral while attempting to count downwards from 20 to 10. ‘Partial speech arrest’ was declared when a subject produced at least one inadequate or semantically changed numeral. The time taken to produce any response was not assessed. The classification was adapted from Thiel et al. [59]. If no effect of stimulation was observed, it was classified as negative.
Participants assessed the stimulation effect and their comfort level using a visual analogue scale (VAS) that we proposed [24]. On the VAS scale, 0 indicated no effect of speech arrest and no comfort while 10 represented maximal speech arrest effect, maximal comfort. Finally, participants indicated the subjective reason for speech arrest, choosing between ‘cannot say a word’ or ‘cannot recall a word’.
Statistical analysis
We performed the χ2 test or Fisher’s exact test (for 2 × 2 contingency table) as appropriate for categorical variables. To compare interval data, we used the Mann-Whitney U test or Kruskal-Wallis test with post-hoc Dunn’s tests, as the data did not follow a normal distribution. Participants were divided into subgroups based on Handedness Deciles [39] (as shown in Table 4) to find the approximate cut-off value of handedness for increased involvement of particular hemisphere in speech processing. Logistic regression analysis was conducted to evaluate potential factors influencing speech processing, including age, sex, handedness, MT and maximal stimulator percentage. A backward stepwise multiparametric logistic regression followed and included all variables with a p < 0.20 from the univariate analysis. Spearman’s rank correlation coefficients were calculated to assess rank agreement of the results between the two groups as it is less affected by outliers [60] and can effectively capture the magnitude of monotonic relationships beyond linear associations [61].
Receiver operating characteristics (ROC) analysis allowed for an assessment of the ability of the LI and Handedness Decile to predict the involvement of hemispheres in speech processing by positive effect of speech arrest – either predominantly over the left hemisphere or predominantly over the right hemisphere (including bilateral localization), exclusively over the left hemisphere, exclusively over the right hemisphere and bilaterally. The area under the ROC curve (AUC) was used to evaluate the predictive value of the Handedness Decile for hemisphere involvement in speech processing, with its 95% confidence intervals. The Z-statistic tested for significant differences in AUC values (H0: AUC ≤ 0.5; H1: AUCN > 0.5). We calculated the optional cut-off value according to the Youden index (J) representing the point of highest accuracy with minimal false-negative and false-positive results. Corresponding negative predictive values (NPV) and positive predictive values (PPV) were reported with their 95% confidence intervals.
Statistical significance was set at p < 0.05. All statistical analyses were performed using StatSoft Statistica v10.0 and Analyse-it for Microsoft Excel v3.53 software.
RESULTS
Speech arrest was successfully induced in 56 participants (96.6%). Regardless of handedness, 53.6% of participants showed a positive response after the rTMS over both the right and left hemisphere, 42.9% exclusively over the left hemisphere and 3.5% only over the right hemisphere. In two right-handed volunteers no speech arrest was induced over either hemisphere, even with a magnetic stimulus of 1.5 × MT, and further simulation the beyond 1.5 × MT was not pursued. 61% of right-handed individuals presented speech arrest after rTMS bilaterally while 39% only over the left hemisphere. No right-handed participants demonstrated speech arrest after rTMS over the right hemisphere alone. No right-handed participants demonstrated speech arrest after rTMS over the right hemisphere alone. Notably, right-sided lateralization occurred exclusively in left-handed participants (15%). Among left-handed individuals, 62% displayed speech arrest only after the left hemisphere stimulation, while 23% showed bilateral effect. Ambidextrous individuals exhibited exclusively bilateral speech arrest. Detailed results are summarized in Tables 2, 3 and 4. The overall concordance rate for positive effect after stimulation over the left and right hemispheres was 55.1%, with a positive concordance rate of 51.7% and negative concordance rate of 3.4%.
Table 2
Participants’ characteristics due to handedness and localization of the positive speech arrest effect*
Table 3
Participants’ characteristics due to localization of the positive speech arrest effect and handedness*
Table 4
The characteristics of the type of positive speech arrest effect due to its localization and handedness*
a – when positive effect exclusively over the left hemisphere compared with positive speech arrest tests for the left hemisphere in a group of people with bilaterally
The prevalence of partial and full speech arrest differed significantly between participants with positive effects exclusively over the left hemisphere and those with bilateral positive speech arrest. The difference was significant for the right-handers but not for the left-handers. Right-handers with speech arrest solely after the left hemisphere stimulation were 10.71 times (OR: 1.31-87.89) more likely to experience full speech arrest when compared to those with bilateral effects (Table 4). Full speech arrest after all stimulations occurred significantly more often in the right-handers than in the left-handers (67% vs. 28%, p = 0.006).
The predictive value of the handedness decile (Figure I) for particular hemisphere involvement in speech processing was analyzed using ROC curves (Figure II). Handedness could predict positive speech arrest after rTMS over the opposite hemisphere (AUC = 0.80, p ≤ 0.001 and AUC = 0.88, p ≤ 0.001). The best proposed cut-off value for increased chance of inducing speech arrest only over the left hemisphere was the 1st right-handedness decile with a sensitivity of 75.9%, specificity of 75.0%, and PPV of 98.0%. The best proposed cut-off value for the increased chance of inducing speech arrest exclusively over the right hemisphere was the 4th left-handedness decile with a sensitivity of 100% and specificity of 87.5%, and PPV of 22.0%.
Figure I
Localization of the effect due to handedness decile values according to Oldfield (Oldfield 1971)
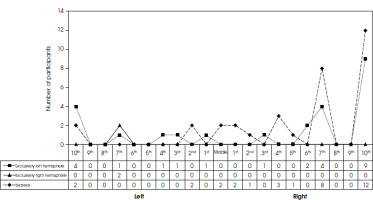
Figure II
A) Evaluation of the Handedness Decile Value as the predictor for speech center activity B) The best proposed cut-off value for the increase of a chance to localize speech center: in a left hemisphere is 1st right decile of handedness with sensitivity of 75.9% and specificity of 75.0%, PPV is 98.0%; exclusively in a right hemisphere is 4th left decile of handedness with sensitivity of 100% and specificity of 87.5%, PPV is 22.0%
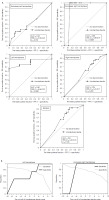
Self-assessed LI did not correlate with inhibition effects, as measured by VAS scale, for neither the left (r = 0.112, p = 0.430), nor the right (r = 0.133, p = 0.349) hemisphere. LI also showed weak, non-significant correlations with participants’ self-assessed comfort during stimulation (left: r = 0.060, p = 0.678; right: r = –0.176, p = 0.222).
Handedness and LI did not correlate with motor threshold (MT) for the left hemisphere (r = 0.074, p = 0.585) or right hemisphere (r = 0.414, p = 0.680), nor with the percentage of stimulator output used to induce a positive effect of stimulation (left: r = –0.511, p = 0.611; right and r = –0.698, p = 0.488).
Self-assessed stimulation effect did not correlate with comfort for either left (r = –0.269, p = 0.119), or right hemisphere (r = –0.067, p = 0.708). Neither motor threshold nor the final stimulator output used to generate speech arrest correlated with the degree of subsequent speech arrest. However, increasing percentage of the stimulator output used to induce speech arrest, significantly reduced stimulation comfort for the left (r = –0.354, p = 0.037) but not for the right (r = –0.078, p = 0.654) hemisphere.
Participants who exhibited speech arrest exclusively over the left hemisphere tended to report higher self-assessed VAS scores of stimulation effects compared to those with positive bilateral speech arrest. However, self-assessed mean comfort, as measured by VAS, was comparable between the groups, as were the mean percentages of stimulator output required to generate the effect (Table 5). Univariate logistic regression analysis revealed no significant factors influencing hemisphere involvement in speech processing.
Table 5
The characteristics of participants’ self-assessment due to localization of the positive speech arrest effect and handedness*
DISCUSSION
To the best of our knowledge, this is the largest study of its kind [32, 36, 55, 62-65] and one of the few ever- performed exploring language and handedness using rTMS [34].
The results of our study suggest that both brain hemispheres participate in speech production, as supported by recent studies [3], contrasting with the traditional view of unilateral left-hemispheric control [4]. In most participants, rTMS elicited speech arrest over both the left and right hemispheres, indicating bilateral involvement in speech production. While this is not entirely new one – neuroimaging studies using fMRI and magnetoencephalography have similarly shown bilateral involvement in various aspects of language processing [66-68] – our study adds further evidence.
The prevalence of bilateral speech arrest is supported by findings by Cogan et al. [69], who identified a bilateral sublexical speech sensorimotor system as well as Hickok et al. [70] and Price et al. [66] who demonstrated bilateral activity in neuroanatomy of speech processing. The involvement of the right inferior frontal cortex in language processing has been observed in both aphasic patients and healthy individuals [71]. Growing evidence suggests that both hemispheres may play comparable roles in language processing in the healthy brain [72]. The right cerebral hemisphere significantly contributes to language processing [73]. It plays a critical role in various aspects of language processing, particularly in prosodic and paralinguistic elements of speech, such as emotional tone, pitch, stress, and rhythm that convey meaning beyond the literal word content. While the left hemisphere is traditionally associated with propositional language – generating novel, grammatical sentences – the right hemisphere plays an essential role in the production and comprehension of nonpropositional, formulaic speech, and narrative interpretation of discourse. It also participates in speech reception, and interpretation, including prelexical, lexical and postlexical components of visual word recognition [73].
Damage to the right hemisphere can impair the emotional modulation of speech, resulting in emotionally flat, monotone speech, as well as difficulties in understanding sarcasm, humor, and other forms of figurative language that rely on context and prosody. The right hemisphere is crucial in integrating and contextualizing linguistic information, enabling nuanced communication [73].
It remains unclear whether individuals with bilateral language dominance are less susceptible to speech decline [74] or the effects of stroke and aphasia. The right hemisphere may either facilitate [71, 75] or impede language recovery following damage [73, 76] to the left inferior frontal gyrus, raising intriguing possibilities for neurorehabilitation [17].
Speech and language abilities have varied components, and the utilization of right hemisphere language pathways does not always hinder the recovery of certain functions [76]. For instance, a study employing diffusion tensor imaging has demonstrated that the integrity of right hemisphere white matter correlates with speech fluency in individuals with chronic post-stroke aphasia [75]. Consequently, we believe that the involvement of right hemisphere white matter pathways in post-stroke aphasia varies and is closely linked to the particular function and anatomical structure involved [76]. More research is needed, however, on speech activity in left-handers [10, 34, 77].
In our study, 5% of participants exhibited speech arrest exclusively after stimulation of the right hemisphere. Khedr et al. [34], study this finding was replicated for 10.5% of individuals. This observation sheds some light on the mechanisms aphasia but explains only a small proportion of cases involving ‘crossed aphasia’ due to damage to the cortex of the right hemisphere [78]. Research on speech lateralization and speech processing inhibition may lead towards greater understanding of recovery from aphasia due to unilateral brain lesions, while it may also result in new rTMS-based therapeutic strategies.
We observed that full speech arrest was significantly more common when it occurred exclusively after the left hemisphere rTMS stimulation (Table 4). In right-handers, the type of speech arrest differed significantly depending on its localization, with full speech arrest occurring significantly more often after left hemisphere stimulation than in those with bilateral effects (p = 0.012).The objective assessment was consistent with participants’ self- assessments of the power of inhibitory effect of the stimulation. The effect tended to be higher in the participants with the effect of speech arrest exclusively on the left when compared to bilateral.
Full speech arrest was also more common in right-handers than in left-handers, and left-hemispheric unilaterality was linked with nearly 11- fold higher likelihood of full speech arrest after rTMS (Table 4), in line with the observations by Knecht et al. [16]. They claimed that, even if individuals with bilateral language dominance still have one dominant hemisphere [79], and the right-hemisphere language network seems to be relevant to aphasia recovery following left hemispheric stroke [51]. Neural plasticity may essentially contribute to language development by allowing non-dominant hemisphere to compensate when the dominant hemisphere is affected by brain lesions [80]. The understanding of the structure and function of speech processing neuroanatomy may provide the basis for successful rTMS therapies [81].
This is in line with the hypothesis of inhibition having a greater impact on speech processing when its network is more active in the left hemisphere. In bilateral localization, unilateral inhibition will cause partial speech arrest. Similar findings in support of this hypothesis were presented by Knecht et al. [10], and Devlin and Watkins [49]. In their work, strongly lateralized subjects were more severely affected by unilateral TMS stimulation than those with bilateral language organization. Pre-morbid differences were observed, which rendered the right hemisphere more or less receptive for language before any re-organization takes place. This may be crucial in determining the likelihood of right hemisphere compensation following left- sided damage.
Future studies should further investigate whether individuals with bilateral speech processing are less affected by ischemia and less prone to post-stroke aphasia. This hypothesis has been previously tested by Knecht et al. [16] who claimed that subjects with weak language lateralization (more bilateral) were less severely affected by left or right-side TMS than those with strong lateralization. They proposed that in case of unilateral damage to the IFG, weak lateralization permits for language function compensation as the spared hemisphere overtakes the function of the affected side.
Our results confirm the link between language lateralization and handedness. The classic theories assume that they are determined by the same ontogenetic factors [3]. Firstly, we observed that predominantly bilateral speech processing is common in most right-handers and all mix-handers. This localization overreaches to the left hemisphere in right-handers, unlike in the previous studies where right-handers were found to be predominantly left- hemisphere-dominant [34]. Previous studies reported bilateral predominance in ambidextrous individuals [34], but in our study, all mix-handed participants had possible bilateral localization of speech processing. While we found no direct correlation between handedness and speech localization, a higher LI and handedness decile in right-handers increased the likelihood of , the left-hemisphere dominance. This aligns with Broca’s original observation [4]. An exact cut-off value can be found for this rule as over the 2nd right decile there is a greater chance to find the activity after stimulation of the left side.
As only two left-handers (both from the 7th left decile of handedness) in our study exhibited speech arrest exclusively after the stimulation of the right hemisphere, it supported the findings that right-hemisphere language dominance may increase linearly with the degree of left-handedness [10] and may be characteristic for left-handers [82]. This is another proof of strongly marked lateralization. The degree of handedness and its impact on speech arrest localization has been discussed in the background of other potential predictors of language center activity. The results of our study, unlike of the previous reports, did not prove the independent role of age [83], sex [84, 85], individual’s motor threshold or maximal stimulator percentage used in speech processing localization predicting. The homogeneity of our group might have influenced the result. The results concerning the effect of age may be in fact biased by the age structure of the group. Szaflarski et al. [44, 83], highlighted the plateau of hemispheric lateralization in young adults so the fact that we recruited young adults only, may have an impact on the results.
The novelty of our procedure lies in incorporating self-assessment of the speech arrest effect [24]. We believe that the participant’s perspective offers a unique insight, and it is of importance in neurophysiological cognitive studies and those bearing any medical indications. We used the VAS which is regularly used in pain assessment. Participants marked the strength of the simulation effect on a 0 to 10 cm scale. To consider the safety/efficacy ratio, we asked participants about the discomfort level during stimulation.
Technological advancements have offered new tools in neuroanatomy of speech processing and functional studies. Modern solutions such as rTMS brought new opportunities [86], and we aimed to leverage these advances by refining tour method and incorporating recent data.
The study is not without its limitations. The key concern is the method of speech arrest induction. We provide detailed elaboration on the methodology used in the study in our previous paper [24]. However, given the widespread and powerful nature of the suprathreshold stimulation applied, we cannot exclude the possibility that, for some participants, the observed speech arrest may have been caused by interference in pure motor areas, including the primary motor areas controlling articulatory muscles. The exact target of TMS was not controlled which could explain why many participants experienced speech arrest after the right hemisphere stimulation. It is likely that the mechanisms underlying the speech arrest were mixed, involving motor planning, motor execution, and linguistic processes. Many subjects reported the “Cannot say a word” effect (Table 5) in contrast to the “Cannot recall a word” effect.
By itself, rTMS lacks the necessary foundation to fully support robust conclusions about the direct causal effects of targeted brain structures [87]. The stimulation-related causal effects may not be equivalent to structure-related causal effects due to TMS side effects, such as electric field distribution or due to indirect (network) effects [87]. Nevertheless, we believe that probabilistic statements about causal impact can be made, though we acknowledge that not only language structures may have been affected by rTMS in our study.
Another limitation was the lack of navigated rTMS when conducting this study. As we outlined in our previous methodological paper [24], we were aware of this constrained and were inspired to work towards creating a simplified, accessible procedure. However, it must be acknowledged that the heuristic approach used for coil placement did not account for individual differences in cortical morphology [24].
Additionally, our study has an overrepresentation of left-handed individuals (15%) compared to general population where left-handers typically constitute around 10% [31]. However, participant recruitment among healthy volunteers, who were medical students, were consecutive and blinded to handedness.
Several precautions were taken to minimize potential bias in the study. We used a standardized handedness questionnaire in its original form [39], which increased the comparability of our results [40]. Furthermore, the procedure was conducted on a group of nearly 50 right-handed participants. Our intention was to simplify the existing methods without affecting their effectiveness and results, making them more accessible. Therefore, our choice of visible estimates of motor threshold is justified, as they were previously reported to be highly correlated with EMG. We independently analyzed the video-recorded procedure, preventing misclassification of speech arrest positivity and enabling a more insightful interpretation of the results [58].
Differences handedness classification and the type of speech arrest effect (we specified full and partial types [34]) may have contributed to discrepancies in the involvement of left and right hemispheres in speech processing between our results and other rTMS studies.
The sequential counting task, an over-learned automatic procedure [88], may have contributed to an overestimation of language bilateralism as it is often preserved in aphasic patients [89]. We chose counting which has been particularly convenient for language mapping [88], but we introduced counting downwards to inhibit the currently activated cognitive schema after the production of each digit [90]. This attention-demanding tasks engages working memory and executive processes [91]. It requires suppression of perceptual or memory cues, employing a Supervisory System [92], responsible for monitoring, oversight, and control during automatic attention processes. Like random number generation, counting downwards constitutes a demanding attention task that activates both working memory and executive functions. We selected these tasks because they mandate retention of information, inhibition of routine counting, and internally guided response generation and monitoring [91].
Further analysis of speech dynamics, such as time taken to produce numerals and verbal fluency, may be beneficial in assessing the full impact of TMS stimulation on speech processing.
CONCLUSIONS
Although handedness does not directly correlate with the active localization of speech function, some trends may be observed using rTMS. Individuals with handedness values over the 2nd right decile are more likely to have left hemisphere involvement in speech processing (exclusively or bilaterally). Those who are left-handed over the 7th left decile are more likely to show right-hemisphere language dominance, though this was observed only in left-handers. Ambidextrous individuals exhibited bilateral speech arrest.
Regardless of handedness, rTMS revealed most common involvement of both hemispheres in speech processing. In right-handers with left-hemisphere language predominance, speech arrest occurred significantly more often than in those with bilateral hemispheric involvement, where the effect was partial.
These new data on the relationship between handedness and language processing offer valuable insights into cognitive science and may guide further research on aphasia treatment using rTMS.