Introduction
Since its emergence in December 2019, the COVID-19 pandemic has led to approximately 7 million deaths worldwide, underscoring its status as a paramount public health issue of current times [1]. With a decline in mortality and hospitalizations from acute COVID-19, there is an observed shift in focus toward investigating the post-recovery complications experienced by COVID-19 survivors, especially so-called long COVID. Long COVID, or post-acute COVID, describes a constellation of persistent symptoms experienced by individuals, which are subsequent to the resolution of the acute phase of the infection [2]. These symptoms, lasting for weeks to months, encompass multiple manifestations including fatigue [3, 4], sleep disturbances [5], paresthesias [6] and cognitive impairment [3, 5], with many more that are still uninvestigated. Nevertheless, according to the literature, fatigue is the most common sequela experienced by patients with long COVID [2, 3], presenting as profound and unrelenting exhaustion, significantly impacting daily functioning and quality of life [7]. The underlying mechanisms driving fatigue in long COVID have not been fully described yet, but it is proposed that inflammatory processes triggered by the initial infection may lead to ongoing fatigue through various pathways, including cytokine dysregulation and neuroinflammation [8–10]. Another interesting hypothesis is an involvement of the tryptophan degradation pathway in development of fatigue among COVID-19 patients which highlights that disrupted levels of tryptophan and its metabolites could affect mood and cognitive function [11, 12]. Tryptophan is an essential amino acid that serves as a precursor for several bioactive metabolites, e.g. serotonin, melatonin, kynurenine, kynurenic acid, quinolinic acid and NAD+ coenzyme [13, 14]. Since disturbances in tryptophan metabolism are linked with mood changes and depression [15], it is to be resolved whether their involvement contribute to development of fatigue in patients with long COVID [11].
Ongoing scientific inquiry is aimed at further unravelling the pathophysiological underpinnings of long COVID, with concurrent efforts directed towards optimizing its clinical management [16, 17]. Therapeutic strategies in long COVID rely on the multidisciplinary approach encompassing pharmacological interventions, physiotherapy, cognitive behavioural therapy and supportive therapies, all geared towards ameliorating functional capacity and enhancing overall quality of life [18]. One of the possible interventions that shows some promising results in treatment of fatigue is using UV-free blue light therapy which so far has not been tested in long COVID patients [19].
Ultraviolet radiation (UVR) therapy thoroughly changed the treatment of skin disorders. While having an established place in dermatology [20], a rapid development of UVR regimens is still observed due to the progressive advances in immunology and photobiology [21]. In general, phototherapy employs body irradiation with UVA or UVB, however in past years a form of ultraviolet-free therapy using blue light emitted by LED lamps (light emitting diode) [22] was constantly attracting the attention of both physicians and scientists due to the lack of side effects linked to the UVA/UVB exposure [23]. Additionally, in vitro studies proven that blue light could reduce the cytokine production by dendritic cells and regulate the proliferation rate of keratinocytes and fibroblasts, which has been verified in multiple human trials regarding e.g. psoriasis and acne vulgaris [23–26]. Interestingly, there is accumulating evidence that blue light therapy could be used in treatment of mood disorders [19]. Multiple studies showed that repetitive exposure to blue light alleviates fatigue, daytime sleepiness and increase cognitive function in different patient groups [27–29]. Nonetheless, studies on the efficacy of blue light therapy in treatment of fatigue in long COVID patients are lacking.
Aim
The aim of the study was to evaluate the effects of blue light therapy in treatment of fatigue among COVID-19 survivors.
Material and methods
The study comprised of 43 adults, 7 males (mean age: 45.00 ±11.10), 36 females (mean age: 38.00 ±12.82) who underwent COVID-19 infection confirmed by either RT-PCR or 2nd generation antigen test (material taken from the nasopharyngeal swab). All participants were reporting a subjective feeling of fatigue 4 weeks after resolution of the infection, which was confirmed by a board-certified physician. Other inclusion criteria were: 18–65 years of age and lack of concomitant metabolic diseases (e.g. hypertension, hypothyroidism). Individuals with hypertension, photosensitivity-related conditions (e.g. porphyria, solar urticaria), active or cured skin cancers, taking immunosuppressive or photosensitizing drugs as well as pregnant and breastfeeding women were excluded from the study. The study was performed in the Dermatology, Paediatric Dermatology and Dermatological Oncology Clinic, Medical University of Lodz, Poland.
All participants underwent 10 full-body blue light irradiation using Phlecs Full Body Blue 1.0 (Phlecs, Eindhoven, Netherlands) emitting 453 nm light (irradiance 40 mW/cm2) for 15 min a day for 5 consecutive days each week (2 days of pause). Before starting the study and after the full irradiation regimen all participants were assessed using the Fatigue Severity Scale (FSS), Short Form 36 Vitality Subscale (SF-36) and Dermatology Life Quality Index (DLQI). Additionally, serum samples were taken from 20 individuals who provided additional consent to measure the levels of tryptophan, kynurenine, kynurenic acid, quinolinic acid/78 and serotonin before and after irradiation.
Statistical analysis
Statistical analysis was performed using the Statistica 13 software (StatSoft, Tulsa, OK). Continuous variables were presented using mean and standard deviation. Distribution of the variables was assessed using the Shapiro-Wilk test. To compare differences between groups, the Student t-test or the Wilcoxon signed rank test were used. To study the exact effects of irradiation and eliminate temporal variable the results of measurements over time were subtracted (valueafter – valuebefore) and Δvalue was created/obtained. The correlation analysis was performed using the Spearman correlation coefficient. A correlation coefficient ranging from 0.00 to 0.19 was considered as very weak, 0.20 to 0.39 as weak, 0.40 to 0.59 as moderate, 0.60 to 0.79 as strong and 0.80 to 1.0 as very strong. A p-value below 0.05 was deemed significant.
Results
No significant changes in systolic blood pressure were noted (p = 0.06), while diastolic blood pressure was significantly lower after 10 irradiations (from 81.2 to 78.5 mm Hg, p = 0.03) (data not shown). Considering clinical scales, a significant decrease in FSS (from 47.17 ±15.27 to 33.12 ±17.59, p < 0.01), increase in SF-36 (from 57.10 ±27.67 to 76.07 ±25.77, p < 0.01) and decrease in DLQI (from 7.62 ±6.49 to 3.95 ±5.02, p < 0.01) were observed. Detailed results are presented in Table 1.
Table 1
Changes in clinical scales used before and after irradiation
Scale | Baseline | After | D | P-value |
---|---|---|---|---|
FSS | 47.17 ±15.27 | 33.12 ±17.59 | –14.05 ±17.69 | < 0.0001 |
SF-36 | 57.10 ±27.67 | 76.07 ±25.77 | 18.74 ±22.56 | < 0.00010 |
DLQI | 7.62 ±6.49 | 3.95 ±5.02 | –3.85 ±3.30 | 0.0003 |
Blue light irradiation also significantly increased the serum levels of tryptophan (from 66.77 ±21.24 to 71.24 ±21.27 mmol/l, p = 0.03), kynurenine (from 2.58 ±1.15 to 3.10 ±1.51 mmol/l, p = 0.02) and serotonin (from 240.55 ±81.19 to 274.93 ±99.66 ng/ml, p < 0.01). Interestingly, the serum levels of quinolinic and kynurenic acid were unaffected by blue light therapy and no significant differences between study points have been revealed. Detailed results are shown in Table 2. A statistically significant moderate correlation was found between the kynurenic acid serum concentration and DLQI (Figures 1–3, Table 3).
Table 2
Changes in the kynurenine pathway serum levels before and after irradiation
Table 3
Correlation matrix between assessed parameters
Figure 1
Changes in Fatigue Severity Scale (A), Short Form 36 Vitality Subscale (B) and Dermatology Life Quality Index (C) before and after irradiation. Data presented as means (squares) with standard error (boxes) and standard deviation (whiskers)
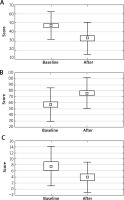
Discussion
Long COVID is associated with a range of neuropsychiatric symptoms, including depression and fatigue [2]. Emerging evidence suggests that the virus may directly impact the central nervous system through neuroinvasion or indirectly through immune-mediated mechanisms, leading to neuropsychiatric manifestations [30, 31]. Fatigue has been reported as one of the most common symptoms among long COVID patients, however the exact pathophysiological mechanisms underlying the development of fatigue are not fully understood [2]. Understanding the underpinnings of fatigue in COVID-19 survivors is essential for comprehensive patient care and developing targeted interventions.
Ongoing research results show that blue light therapy may be effective in alleviating fatigue among patients with cancer [29] or depression [27], as well as in reducing depressive symptoms, particularly in individuals with seasonal affective disorder [32]. While the precise mechanism of action is unclear, it is hypothesized that blue light therapy alters serotonin secretion, suppresses melatonin production and modulates circadian rhythm via retinothalamic tract/suprachiasmatic nucleus [32–34]. Moreover, blue light therapy has minimal side effects compared to antidepressant medications, making it an appealing option for patients seeking non-pharmacological treatments. In our study a significant decrease was observed in both FSS and SF-36 scales, showing that repeated exposure to blue light may decrease the subjective feeling of fatigue which requires further investigation. To the best to our knowledge this is the first report describing successful use of blue light therapy in treatment of fatigue in long COVID patients and remains consistent with current literature data regarding usage of blue light in treatment of fatigue. Srisurapanont et al. [19] performed a comprehensive analysis of the effectiveness of blue light therapy in alleviating daytime sleepiness, sleep disturbance, depression, and fatigue in patients with post-traumatic brain injury (TBI) [19]. The study revealed that exposure to blue light may have a beneficial effect on reducing feelings of tiredness and exhaustion commonly experienced by individuals with TBI, while having almost no significant effect on sleep disturbances [19]. Similar results were provided by Wu et al. [29] for breast cancer survivors in whom exposure to bright blue-green light decreased fatigue and sleep disturbances experienced [29]. While not widely tested in different patient cohorts our results suggest that blue light could be used as adjunctive treatment of fatigue in long COVID patients.
Another interesting finding provided by our study is a significant increase in tryptophan, kynurenine and serotonin, without changes in quinolinic and kynurenic acid serum levels after the therapy regimen, thus implicating that repeated exposure to blue light may affect the tryptophan metabolism and degradation pathway. The initial step of tryptophan degradation involves the conversion of tryptophan by either indoleamine 2,3-dioxygenase (IDO) or tryptophan 2,3-dioxygenase (TDO) to N-formylkynurenine, which is rapidly converted to kynurenine by the enzyme formamidase [35]. Kynurenine is the central metabolite in the tryptophan degradation pathway and can undergo further metabolism through two main branches: the kynurenine pathway and the serotonin pathway [35]. In the kynurenine pathway, kynurenine is metabolized by kynurenine hydroxylase to form 3-hydroxykynurenine, which is further metabolized to kynurenic acid, quinolinic acid, and picolinic acid [35]. Alternatively, kynurenine can be converted into serotonin via kynurenine hydroxylase and aromatic amino acid decarboxylase [14]. As shown by Al-Hakeim et al. [12, 36] chronic inflammation disrupts the kynurenine pathway which may contribute to development of fatigue in long COVID patients [36]. Specifically, a decrease in tryptophan along with an increase in kynurenine has been found to be associated with fatigue experienced by COVID survivors [36]. These findings are supported by Maes et al. [37] who linked somatization disorders with tryptophan depletion and kynurenine increase [37]. Under physiological conditions kynurenic acid acts as a neuroprotective compound by blocking N-methyl-D-aspartate (NMDA) thus inhibiting glutamate signalling [38]. On the contrary, quinolinic acid acts as an agonist of the NMDA receptor, leading to increased glutamate activity which eventually leads to neuronal loss by promoting oxidative stress [39]. Taken together, our results suggest that blue light therapy may increase serotoninergic transduction and does not contribute to increased levels of the tryptophan pathway metabolites associated with neurotoxicity (quinolinic acid), but overall should be taken with caution. The presented study has several limitations. First of all, the patients should have been screened for depressive symptoms since one of the most common causes of fatigue is undiagnosed depression. Secondly, while obtained results show that blue light therapy could be used as adjunctive treatment of fatigue in long COVID patients, another study with a placebo group is needed to establish a possible causal explanation for these phenomena. Lastly, more robust research is necessary to establish the effectiveness of blue light in alleviating fatigue with special attention given to longer study duration and larger group sizes.