Introduction
Penetration of microplastics into living organisms may occur through ingestion, inhalation or direct contact with the skin, but the main way of absorbing this type of contaminants seems to be its ingestion [1,2]. For example, Cox et al. [3] found that Americans consumed an average of 39,000 to 52,000 particles of various microplastics per year, while for those who drank water from plastic bottles, the number of particles rose to as much as about 90,000/year. The most abundant sources of microplastic consumption are so-called seafood, such as clams, shellfish and fish, as well as sugar and salt [2]. Thanks to the analysis of microplastics in the bodies of mammals and humans, it was observed that only 0.3% of microplastics can cross the epithelium of the gastrointestinal tract and enter the human body, and their size is approximately 150 μm. It has also been observed that of these 0.3% microplastic particles, 0.1% are larger than 10 μm, and thus should have the potential to reach cell membranes and organs by passing through the placenta and blood-brain barrier [4].
Plastics are a diverse form of synthetic polymers with a wide range of size, shape, composition, properties and application potential. Microplastics (MP) are defined as small plastics with a particle size of less than 5 mm, and microplastics with a size of less than 0.1 μm are called nanoplastics (NP). In addition to primary microplastics used in personal care and household products, secondary microplastics are commonly found in the environment due to the degradation, transformation and fragmentation of parent microplastics derived, for example, from used packaging, which is driven by photo-oxidation and physical processes [5,6].
Plastic products are inexpensive, convenient and have many uses in our daily lives, but they are usually difficult to degrade and recycle effectively. Plastic food packaging can eventually break down into pieces of plastic and contaminate food products. Microplastic particles have been detected in almost every environment affected by human interaction, including aquatic, terrestrial and atmospheric environments [7-9]. It has been reported that microplastics have negative effects on living organisms by inhibiting their growth, development and reproduction [10,11]. However, there is an extensive knowledge gap about microplastic pollution and its impact on human health. Detecting and quantifying MP in important areas of our lives, such as nutrition, is difficult due to the lack of reliable analytical methods. Another challenge for researchers is to understand the physicochemical properties of polymers, which are typically heterogeneous and pose a major challenge in making appropriate toxicological assessments.
Recently, reports of possible microplastic contamination of bottled water have been published [12]. Given the high consumption of bottled water around the world, the topic is attracting widespread media attention. Mason et al. [12] concluded that some of the contamination in drinking water comes from the water source, while most of the contamination comes from the packaging. They showed that there is less fiber in tap water than in bottled water, indicating different sources of drinking water contamination. The authors found secondary contamination of water, inside the bottle, resulting from the fragmentation of the cap during the opening and closing of the bottle. The researchers published these results in 2018, using Nile Red staining (for particles >6.5 μm) and further identification by FT-IR (for particles >100 μm) as a method for detecting microplastics [12]. Also in 2018. Schymanski et al. [13] analyzed microplastic contamination of bottled water from single-use and returnable plastic bottles, cartons, and even glass bottles from Germany. The sample size was smaller than that of Mason et al. [12]. Here, the researchers used μ-Raman spectroscopy and were able to identify particles smaller than 20 μm. In this study, the researchers found a high number of MP particles in disposable bottles, but also in returnable bottles (84% of the particles were polyethylene terephthalate, PET, and 7% were polypropylene, PP, which are the main components of plastic bottles). Polyethylene and other polyolefins have been found in water from beverage cartons and also from glass bottles. The authors attributed the presence of these MPs in water to beverage carton coating materials and polymers from bottle caps [13]. It seems that plastic contamination varies – depending on the combination of polymers (mainly PEST and polypropylene), and the size of the particles selected for the study varied, due to the different methodologies used in the two study groups (>100 μm or >6.5 μm). The results of the study clearly indicate that packaging can release microplastic particles into drinking water [12,13]. Another study on the release of MP particles from the bottle neck and cap material after the procedure of repeatedly opening and closing bottles was conducted by Winkler et al. [14]. Samples of bottle caps from bottles opened and closed 100 times showed impressive signs of mechanical wear, such as abrasions and deep grooves. In this case, the release of MP particles from disposable PET mineral water bottles was considered after exposure to mechanical stress using SEM and EDS. This allowed for morphological and elemental analysis of the plastic surface and quantification of the concentration of MP particles in the water sample. The goal of this study was to better assess the sources of MP uptake from plastic bottles, especially considering the impact of daily use of these bottles, such as abrasion of the plastic material that builds their components. These authors tested the release of MP from PET bottles and HDPE caps on their surfaces after a series of bottle openings/ closures. They investigated whether the inner surface of PET bottles releases MP, counted and identified MP in bottled water. The results showed a significant increase in the occurrence of MP particles on the surface of PET and HDPE material (bottles and caps) after repeated mechanical opening and closing of bottle caps [14]. Even an analysis of our daily tea bags revealed that tea drinkers consume billions of microplastic (MP) particles from a plastic tea bag. Although the tea analysis included MP particles 50 times smaller than those measured in water, a clear method for quantifying microplastic in these studies is not available [15].
Food and beverages are the main carrier of microplastics and nanoplastics, through which they are transported into living organisms [1,2,16]. The use of antimicrobial food packaging materials, including additives such as titanium dioxide and carbon nanotubes, has shown that the effect is comparable to the use of nanopolymers [17,18]. Mixtures of plastic additives can dissolve in the polymer and leak into the surrounding environment [19]. In addition, metals (i.e. Ag, Cd, Co, Cr, Cu, Hg, Ni, Pb and Zn) can be accumulated along with microplastics in fresh water [20]. It can be seen that the deleterious effects of plastic at the nano- and micro-scale may not be limited to the physicochemical properties of the plastic itself, but may also be attributed to co-occurring chemicals, such as additives and absorbed chemicals from the surrounding environment [21-23]. For example, the so-called “Trojan horse” principle, a mechanism by which plastic particles can serve as vectors to transfer other, often very harmful, chemicals [24]. The rate of absorption, excretion and toxicity of microplastic contaminants depend on many factors (age, gender, disease, genotype, physical activity and many others). Since there is little data to date on the occurrence of MP in human tissue samples, modeling studies are needed, which can provide the best estimate of contaminant concentrations in tissues and may be useful in future studies of the effects of microplastics on human health [25].
Aim of the work
The purpose of the study was to determine the rate of wear of the plastic grinder’s burrs and the amount of plastic released from the packaging in which black peppercorns and Himalayan salt are packaged and ground.
Material and methods
Types of samples
The research on the wear of plastic grinding mechanisms was carried out on a sample of five types of spice grinders, which were designated as: A, A’, B, B’, C, D, and E (Figure 1). The research material was purchased from Polish grocery stores. The containers tested contained spices from four of Poland’s leading spice distributors and manufacturers. One spice distributor has two different types of grinders in its product line (B, B’ and C), which are sold under two brand names. All grinders are built with a glass container for storing the spice, which ends in a plastic mechanism containing the grinding burrs along with a lid. Already preliminary studies of the mechanisms using FTIR have confirmed that they are made of a mixture of polymers along with additives. In one type of the tested grinders containing both black pepper and Himalayan salt (A and A’), it is possible to choose one of three degrees of grinding of the product, and in type D there is a grinding adjustment system from maximum grinding to minimum grinding, where we get large pieces of spices after grinding. During the tests, grinding was analyzed at the average level in the case of three adjustable fractions and in the middle setting, between MAX and MIN – which allowed to obtain grinding similar to other grinding mechanisms. In the two types of grinders (A, A’ and B, B’), the spices to be ground were both black peppercorns (A and B) and coarse Himalayan pink salt (A’, B’). The grammage in each grinder varied. For black pepper, it was respectively: A. 42 g, B. 36 g, C. 38 g, D. 47 g, E. 50 g a for Himalayan salt: A’. 85 g and B’. 88 g.
Sample preparation and filtration
Spice grinding was carried out under laboratory conditions with possible conditions for separating the grinding mechanisms as well as the ground material from microplastic contamination from both the air and the surfaces of furniture or utensils used in the tests. All the vessels used in the experiment were glass with tight seals. Analyses were performed in triplicate in three stages:
0% – initial stage of burrs – new burrs before grinding,
50% – used up half of the content determined by weight/volume,
100% – final after grinding the entire composition of the packages.
Grinding was performed by one person in a uniform, measured manner, using similar force and under identical conditions for each type of grinder and its repetitions.
The grinding mechanisms of the grinders were disassembled and analyzed in the smallest possible parts (Figure 2). Tests were conducted on 21 grinder, 3 each for each spice, resulting in 7 test groups: A, A’, B, B’, C, D and E. Then, for each of the 21 grinders, the plastic mechanisms were examined and weighed in 3 repetitions as: new (0%), after the contents had been ground to half their original volume (50%) and after all spices had been used up (100%).
This made it possible to determine the percentage consumption of grinding parts of burrs made of specific polymers for different types of grinding parts. For individual grinders from Polish producers, the rate of wear and tear along with the grinding process and the average loss of burrs expressed in grams in two phases of 50% and 100% of grinding the spice material were also expressed.
By rotating the contents of the grinder three times, approximately 0.1 g of pepper was etched with NaClO/KOH (1:1) [26] for several days to eliminate the background of organic particles, and then the solution was filtered on G8 glass fiber filter discs (Fisher Scientific) using a syringe. Filters were stored in sealed glass Petri dishes to avoid cross-contamination and analyzed using an FT-IR IRT-100/AIM-9000 spectrophotometer (Shimadzu). The most important polymers as the main components of grinders are registered in polymer spectra based on data in the Shimadzu database. Under SEM microscopic conditions (HITACHI 4700) using magnifications: 40, 50, 80. 100, 150, 200, 250 and 500, the smallest possible parts of the plastic grinding mechanism were observed from these three stages of burrs wear.
Results
Grinding as a mechanical process depends on: the grinding material, the hardness of the burrs and ground material, and the size of the grinding products. Grinder components must withstand abrasive forces and stresses. This study can be considered preliminary, as what is needed is to get into the structure of the plastics that build the plastic components of grinding elements for salt and other condiments, and to study their effects on the health of people who consume a very complex number of microplastics with food, but also take in such contaminants from the surrounding environment. Several other studies conducted in this problem area have shown that different types of spice grinders can emit different numbers of complex polymer particles, mainly particles such as: PET, PMMA, POM and PS [27].
Our research shows that not only the coarse pink Himalayan salt contained in the grinders causes significant wear on the plastic grinding mechanism, but also such a spice as whole black peppercorns causes abrasion of the polymeric part of the grinders’ burrs (Figures 3 and 4).
Figure 3
Wear of plastic grinder burrs
Notes: Calculated as a percentage of the initial weight of the grinder, at two stages of consumption: half consumption of 50% and after grinding the whole 100%, black pepper grind A: A, B, C, D, E and Himalayan salt A’ and B’. The whiskers marked on the graph indicate the standard deviation (SD).
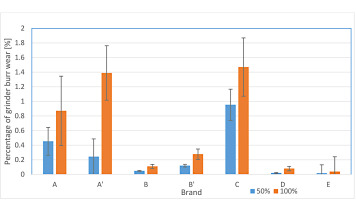
Figure 4
The wear rate and the amount of microplastic released (in grams) from the burrs of each grinder during spice grinding
Notes: The points marked on the graph are the measured data, while the segments between the points are the consumption prediction.
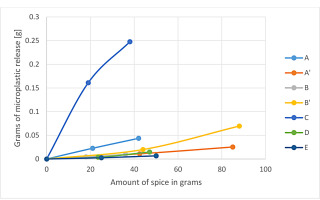
For laboratory tests aimed at determining the quantitative contamination of food by the components of spice grinders, grinders containing Himalayan salt turned out to be easier due to its solubility in water. Grinding whole black peppercorns required laborious separation of the contents from microplastic particles after the etching and filtration process. All groups of grinders were taken in repetition for the study and their grinding mechanisms were weighed in 3 stages: original (0%), half-used (50%) and fully used (100%) after grinding the entire spice content. Type A, A’ and B, B’ have a similar polymer blend in composition, made mainly of: POM, URETHAN and PU (Figure 4). Polymer composition conditions were analyzed and identified by FT-IR from the highest probability of POM content to the lower of the percentage composition of other components according to SHIMADZU Polymers Library. The wear of plastic grinding mechanisms has been shown to depend on both the spice and the composition of the polymeric grinding parts. In the case of type A (black peppercorns) and A’ (Himalayan salt) grinders, their wear rate is greater when grinding pepper. In contrast, the opposite results were obtained for the B and B’ grinders, where Himalayan salt resulted in higher wear and, with it, a greater amount of microplastic released (Figures 3 and 4).
In a study of the wear and tear of the grinding parts of various types of plastic spice grinders, special attention was paid to their polymer composition using an FTIR spectrometer (Figure 5). It turned out that after the contents had been completely ground, the grinder named C in the project, made of nylon as the main polymer, wore out the most (Figures 3 and 4). Type C grinders have a black grinding mechanism, and this polymer blend is the weakest of the entire test group, meaning Type C loses the most black plastic particles. Currently, the GIS, based on research by the State Sanitary Inspectorate, warns against using black plastic kitchen spoons and potato kneaders made of black nylon for food preparation [28]. Analyses have shown that during contact with food, toxic compounds can enter food in amounts that can pose a health risk to consumers [28]. Comparing the rate of wear of polymeric burrs in different types of grinders from specific manufacturers, the highest wear was observed for the C grinder, followed by the following: A, B’ and A’-the latter two with Himalayan salt. The dynamics of change are illustrated in Figure 4, which shows the average weight loss of the grains due to grinding for two amounts of ground spices 50% and 100%, which corresponds well with the previously described durability of the other types of grinders (Figures 3 and 4).
Figure 5
Spectra of the main polymers found in grinder burrs according to SHIMADZU Polymers Library, before their use Notes: There is a dominance of several polymers identified by FT-IR: POM (a), PMMA (b) and PC (c).
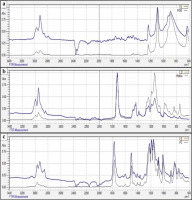
Based on the weight determinations of the grinders’ wear in 3 subsequent stages of use, it turned out that the grinders contain the main polymers in the order of their composition: POM, URETHAN, PU (types A and B) are more resistant to abrasion and wear much less than type C, whose main building material is nylon (Figures 3 and 4).
Next in the ranking of hardness and durability are E-type grinders with plastic parts, represented mainly by PC and Epoxy (Figures 3, 4 and 5b). Polymer-containing grinders proved to be the most durable and suitable for grinding spices when the library of FT-IR spectra suggested the highest contents of PMMA and PVC (denoted as D) in their composition (Figures 3, 4 and 5).
The different degrees of abrasion of the materials that build the grinding parts of the grinders are due to the hardness of the polymer mixture, as well as the spice being ground. Plastic parts wear during grinding, which was visualized at a magnification of 40 to 500 times using SEM microscopy (Figures 6 and 7).
Figure 6
Plastic mechanisms of type A grinder using SEM microscopy
Notes: new grinders (a), after grinding half of their contents 50% (b) and after all spices have been used 100% (c).
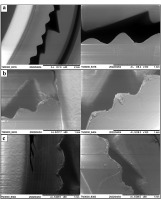
Figure 7
Plastic mechanisms of type B grinder using SEM microscopy
Notes: new grinders (a, b), after grinding half of their contents 50% (c) and after all spices have been used 100% (d).
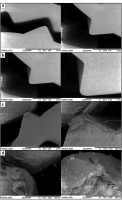
Figures 6 and 7 show images of the grinders of manufacturer A and B which are the most representative and allowed to take in the best quality images. Limitations were due to the peculiarities of SEM microscopy, spherical limitations, the size of the elements under study or the sharpness of the images obtained, among others.
Discussion
The problem of microplastics in the environment and food is widespread. According to a study conducted by a team of researchers from the University of Newcastle in Australia, up to 5 grams of microplastic enters the human body per week, mainly through the oral route [29].
Several analytical methods are most commonly used to detect and characterize microplastic particles in food, e.g.: FT-IR, Raman, SERS, SEM, TEM.
In this study, the abrasion of plastic particles constituting burrs in Himalayan salt and black peppercorn grinders was investigated using FT-IR and SEM techniques. They were suitable for our experimental set-up because they provided high-resolution images of the surfaces of plastic grinding mechanisms and revealed the main building block component. This compilation of techniques was used to detect attrition and determine the release of essential types of microplastic particles into food along with ground spice particles.
Similar research was conducted by a team led by D. Schymanski. They noted the release of microplastic particles when using salt or spice grinders using FT-IR and μ-Raman spectroscopy methods [27]. They verified that the PMMA polymer is harder than PS, which is in turn harder than POM. Most of the PET polymer particles from the grinding system were smaller than 5 µm, while POM, PS and PMMA had average sizes larger than 10 µm. Microplastic contamination was highest when the grinding burrs were made of POM. Other polymer particles included in the grinders may also get into the spices during grinding, as the polymer compositions of the building blocks are very complex [27].
According to the results, although spice grinders are widely considered safe and widely used for food packaging, they have disadvantages such as the release of microplastics due to grinding and wear of plastic grinding parts.
Concerns about food safety prompted a number of salt and pepper grinders to be recalled in Australia in September 2019. The entire series of grinders had faulty packaging, leading to the potential presence of plastic fragments in the spices [30].
The observed differences in the release of microplastic particles between brands of grinders show a variety of plastic behavior depending on the composition of the polymer used as a building block for the grinders. This is due to the variety of plastic behavior of building substances, which must be taken into account in order to optimize the approval criteria for food contact packaging. The degree of abrasion of this type of product intended for grinding spices depends on the durability of grinding burrs made of various polymers – most often of multiple components. Nylon appears to be relatively the least durable, and the other polymers, in order of durability, are: PMMA, PC, POM – more suitable for this type of kitchen equipment (Figure 3). A similar order is indicated by textbook standards of hardness for these polymers, the determined so-called ball hardness (30s) gives ranges in MPa, respectively, for PMMA 180-200, PC 110-145, POM on average 140 and for nylons, or polyamides in the range of 70-90 [31], which is confirmed by other studies [27]. In addition to these polymers in food, which are obtained by grinding from the plastic spice grinding parts, primary contaminants are possible.
Food-contaminating microplastics have unclear effects on humans and other organisms. It is commonly found in blood, urine or human organs [32-35]. Studies have shown that exposure to plastic microplastics causes intestinal inflammation and metabolic disorders of the liver, but it is not yet known whether the damage and inflammation can cause later development of serious diseases [36-39].
Conclusions
Studies conducted confirm the varying rate and amount of microplastic released, which depends on the material from which the grinders’ burrs are made. The highest amount of microplastic released occurred in the case of a burr made of nylon as the main polymer. The use of this polymer, even in kitchen utensils, has raised concerns among Polish sanitary services. The high rate of microplastic particles released from this type of device, confirmed during testing, as well as the warnings announced, confirm that this is not a polymer composition predestined for this type of device. More suitable for this type of devices are polymers (from the most durable): PMMA, PC or POM.
The second factor determining the amount of microplastic released is the type of spice. Manufacturers usually opt for one model of grinder for different spices. This finds a marketing rationale among other things related to branding and brand recognition. However, the study confirms that the different rate of grinder wear depends on the specific spice. Type A and A’ grinders wear faster when grinding black peppercorns, while Type B and B’ grinders wear faster when grinding Himalayan salt.
The research conducted is pioneering in the spice market in Poland and should be continued. It is worth mentioning that there are no standards for the presence of microplastic in food, which could lead to monitoring its content in food.
Determining the impact of using such devices on human health requires long-term analysis.