Carotid-related strokes, caused by either isolated extracranial carotid artery occlusions or “tandem” (extracranial plus intracranial) occlusions account for 20–30% of ischemic strokes [1]; they are often large and severely disabling [2–4]. Today, there is no doubt that eligible patients should be revascularized on an emergency basis in order to maximize cerebral tissue salvage [4–7]. However, acute carotid-related strokes present significant management challenges due to the large thrombo-embolic load. These challenges include the risk of iatrogenic cerebral embolism on the one hand and haemorrhagic stroke transformation on the other [4, 8, 9].
Emergency carotid artery stenting (eCAS) – in contrast to than leaving the carotid lesion unstented – is associated with a greater likelihood of successful reperfusion and better functional outcomes than no-stenting [5, 10]. An important limitation of the 1st generation (single-layer) carotid stents is that they may fail to insulate the athero-thrombotic material [11, 12]. To minimize the likelihood of new cerebral embolism via the “cheese-grater effect” in single-layer carotid stents, these stents are often suboptimally implanted (undersizing and/or lack of post-dilatation optimization), increasing the risk of stent thrombosis and occlusion, with a negative effect on clinical outcomes [4, 8].
The MicroNET-covered stent (CGuard) consists of a very widely open-cell (laser-cut) metallic frame (free cell area of ~22 mm²; i.e., roughly twice that of Zilver or Acculink) that is wrapped by an outer, single-fiber knitted polyethylene terephthalate MicroNET adaptable sleeve (fiber thickness ~25 μm; cell size ~0.02–0.03 mm2; mesh fixation to the frame on stent edges, Figure 1) [13, 14]. CGuard combines properties of the most open-cell metallic stent frame (and thus very high conformability) with the smallest-cell anti-embolic layer [13, 14]. The MicroNET pore size is similar to that of embolic protection filters, resulting in a dense plaque coverage between the sparse struts [11, 15]. These MicroNET sealing properties [15, 16] provide protection against embolism in case of plague tissue crossing the strut line intra-luminally (Figure 1) [17]. The MicroNET-covered stent shows no foreshortening or elongation and exhibits self-adaptability to the artery diameter (within the device nominal diameter; “SmartFit” characteristics) [13, 14, 18].
Figure 1
Photograph of the MicroNET-covered carotid stent (CGuard). Note a widely open-cell nitinol frame (exhibiting a high adaptability to curves), wrapped on the outside by the MicroNET sleeve.
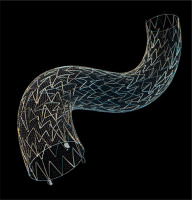
In elective CAS, the MicroNET-covered stent has level-1 (randomized controlled trial) evidence for its profound reduction of intra-procedural cerebral embolism and total elimination of post-procedural plaque-related events [19, 20]. The stent shows normal healing in the absence of any device-related issues in long-term follow-up [21, 22]. Clinical data indicate a significant improvement in 30-day and 12-month clinical outcomes against conventional (single-layer) carotid stents and carotid surgery (≤ 1% death/stroke/myocardial infarction by 30 days, and ≤ 2% combined 30-day death/stroke/myocardial infarction plus any ipsilateral stroke by 12 months) [20–23], transforming the field of carotid revascularization in primary and secondary prevention of carotid-related stroke [1, 24–28]. However, until recently the MicroNET-covered stent had not been systematically evaluated in acute carotid-related stroke including management of “tandem” lesions, with both bleeding and thrombosis risk [4, 9, 29].
The investigator-initiated SAFEGUARD-STROKE Study (NCT05195658) evaluated cerebral and clinical outcomes with routine use of the MicroNET-covered carotid stent (CGuard, Figure 1) in a multi-center and multi-specialty setting, in patients with acute carotid-related stroke eligible for emergency recanalization [30]. Seventy-five consecutive patients were recruited in 6 study centers [30]. Other than the study device use, as per the study protocol, the treatment (including procedural strategy and pharmacotherapy) was performed according to each center’s/operator’s practice [30]. The study found that – despite variability in procedural strategies and pharmacotherapy – the MicroNET-covered anti-embolic stent use in an otherwise routine eCAS was associated with (1) a high angiographic success rate, (2) high 90-day patency and (3) favorable clinical outcomes at 90 days [30].
In the SAFEGUARD-STROKE Study, a cerebral protection device was used in 45.3% of patients. In most cases (38.7%) it was a proximal protection device. This included use of a single balloon (mono-balloon) in the common carotid artery (CCA), dual-balloon (occlusion of both the external carotid artery (ECA) and CCA), and the “dynamic” transcarotid system (TCAR) in 8, 19, and 2 patients, respectively. In two further patients, the balloon guide in the CCA was combined with a distal filter [30]. Filter-only protection was applied in 4% of the study patients [30]. A retrograde strategy (resolving the intracranial lesion first, followed by stenting of the extracranial carotid lesion) [4, 5] predominated (69.2%) in tandem lesions (52% of the study lesions) [30]. The in-hospital mortality rate was 9.4%, and 12.2% at 3 months [30]. At 3 months, mRS0-2 was achieved in 74.3%, mRS3-5 in 13.5%; stent patency was 93.2%. Balloon catheter use for cerebral protection was associated with a reduced risk of bad clinical outcome at 90-days (OR = 0.68; 95% CI: 0.21–0.89; p = 0.003) [30]. Use of a small-diameter balloon for post-dilatation or absence of post-dilatation was an independent predictor of stent patency loss (OR = 15.2, 95% CI: 5.7–73.2, p < 0.001) [30].
We appreciate favorable comments from our Buffalo colleagues on the role of the findings from the SAFEGUARD-STROKE Study [31]. Also, we would like to echo the Buffalo view on the importance of using cerebral protection in eCAS involving the anti-embolic CGuard stent [31]. Cerebral protection is crucial to prevent the carotid lesion-related cerebral embolism that may occur in the eCAS stages prior to stent implantation as the stent–exerted neuroprotection occurs only after stent placement and balloon optimization [3, 11]. In the SAFEGUARD-STROKE Study, all 3 instances (4%) of new cerebral embolism (including 1 with a “direct” stent insertion through a thrombotic lesion) occurred in unprotected eCAS procedures [30].
It is important to understand the limitations of filter protection, including the “unprotected” lesion crossing as well as those arising from the filter malapposition, pore size, and the filter basket capacity [11]. These limitations continue to be relevant in contemporary CAS and eCAS [32]. Thus practical knowledge of how to effectively perform proximal cerebral protection is fundamental for today’s competent CAS and eCAS [11, 33]. Effective proximal protection is only exerted by ensuring absence of antegrade flow in the internal carotid artery (ICA), with aspiration of the debris [11]. Its efficacy can be enhanced by achieving ICA flow reversal throughout the procedure [11, 34]. Experimental evidence shows that the efficiency of proximal occlusion with flow reversal improves with increasing back-pressure (that cannot be influenced other than by volume-loading and withholding antihypertensive medication on the day of CAS) and with repeated aspirations [35].
Proximal cerebral protection offers important advantages in CAS [11, 33] and eCAS [7, 31]. Similar to the Buffalo group [7, 31], we are convinced protagonists using proximal protection in eCAS [3, 29]. However, we noticed that when only occluding the CCA (leaving the external carotid artery patent; CCA mono-balloon use) proximal protection may be significantly impaired, due to persistent flow from the ECA to the ICA – and flow towards the brain in the ICA, rather than ICA flow arrest or reversal (Figures 2, 3) [36]. This phenomenon, which does not occur when the mono-balloon catheter is placed in ICA for distal ICA and/or cerebral thrombectomy in absence of the carotid bifurcation lesion, can be prevented by placement of an ECA flow exclusion balloon catheter (or a ballon wire [37]; regrettably, not currently available). Experimental studies show that endovascular occlusion of the CCA-alone does not sufficiently prevent cerebral embolization [38]. Accordingly, the inability to exclude the ECA (anatomic reasons or severe ECA stenosis) with the (double) balloon catheter system is widely considered a contraindication to using proximal protection in endovascular revascularization of the carotid bifurcation [39].
Figure 2
Example of ECA-to-ICA flow with balloon occlusion of only the common carotid artery (mono-balloon catheter), resulting in lack of effective cerebral protection
A 56-year-old male patient with recent left-hemispheric TIA with severe left carotid bifurcation stenosis (mixed plaque with both calcifications and intraluminal hypoechogenic elements on duplex ultrasound imaging) was treated with 2nd-generation stent CAS for primary stroke prevention. In this setting, proximal protection was considered the system of choice [11, 36]. A mono-balloon system [31] was used because of the significant risk of causing iatrogenic embolism with a double-balloon system (that would require positioning of the distal balloon in ECA) [33]. A mono-balloon proximal neuroprotection system was positioned in the CCA and inflated (A, yellow arrow; “back” pressure 43/38 mm Hg), and antegrade contrast injection (A, white arrows) was performed for subsequent lesion visualization under flow reversal (note the antegrade opacification of the superior thyroid artery in A, red arrowheads). Flow reversal was established by opening the stopcock of the balloon catheter system. Note the disappearance of the contrasted blood in the superior thyroid artery (B, arrowheads) as well as the presence of new uncontrasted blood in the LECA (vessel contour delineated with a dotted line in B). C–F demonstrate progressive, antegrade filling of LICA with uncontrasted blood, indicating flow towards the brain and thus lack of cerebral protection (white dotted arrow in C–F; note also the red arrow in E and F insets – zoom the bifurcation). There is also a degree of retrograde (though non-robust) flow in the CCA, as evidenced by the appearance of uncontrasted blood in the distal CCA; note the dotted vessel contour in F vs. B). Because of lack of any effective cerebral protection with the mono-balloon system in this case, a filter was added (G, white arrow). Under filter protection, the lesion was predilated with a 3.5 mm coronary balloon (G, inset) followed by the MicroNET-covered anti-embolic stent placement (CGuard 10 × 30 mm, H). The stent was sequentially post-dilated with a 6.0 mm non-compliant balloon up to 20 atm and finally by an 8.0 mm balloon up to 20 atm (I), consistent with the stent optimization PARADIGM protocol [13]. The final carotid bifurcation and cerebral result are shown in J and K (the inset is a photograph of the debris captured in the filter in this procedure). Note the optimal endovascular reconstruction of the carotid bifurcation and lack of any signs of cerebral embolism. The patient is part of the PARADIGM-MC (MultiCentric) consecutive patient study.
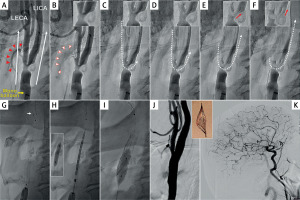
Figure 3
New cerebral embolism in eCAS with balloon catheter effective occlusion of the CCA only and residual flow towards the brain (cf. Figure 2)
A 74-year-old female patient with ‘stuttering’ left hemispheric carotid-related non-tandem stroke (National Institutes of Health Stroke Scale (NIHSS) fluctuating from 3 to 7, Alberta stroke programme early CT score (ASPECTS) 10) was treated with eCAS under proximal cerebral protection. A double-balloon proximal neuroprotection system was inserted in the ECA and CCA; the CCA balloon was inflated (A, green arrowhead; “back” pressure 50/44 mm Hg) while the ECA balloon could not be inflated (A, red arrowhead; balloon dysfunction possibly due to significant calcifications in the proximal CCA in the absence of a significant stenosis); antegrade contrast injection. B shows absence of an intracranial large vessel occlusion (LVO), consistent with prior information from computed tomography angiography. The lesion was predilated with a small coronary balloon followed by insertion of a 9.0 × 30 mm anti-embolic MicroNET-covered stent (C). The stent was implanted and post-dilated (D; 5.0 mm semi-compliant balloon). There was an optimal procedural result at the level of the carotid bifurcation (E) but the patient developed new, severe left hemispheric symptoms. Left-hemispheric cerebral angiogram (F) demonstrated embolic material in the proximal middle cerebral artery (MCA) (red arrowhead) and distal occlusion of the M1 segment (red double arrowhead in F). On-site cerebral mechanical thrombectomy (MT) was performed ad hoc (in the regional Comprehensive Stroke Centre, serving a population of 3.4 million [42], two other patients were already waiting for MT). G shows the introduction of a 5F neuroaspiration catheter; H shows aspiration (catheter tip marked with an arrow; catheter contour enhanced with a dotted line), leading to an effective recanalization of one of the M2 branches (M2a). The other M2 branch could not be opened despite several aspiration attempts followed by SOLUMBRA technique using a small-diameter, adaptable stentriever [29]. J is the final result of the partly-successful MT (green arrowheads show the opened M2 branch; the red arrowhead indicates the remaining M2 branch occlusion, M2b) – and the final angiographic cerebral result. Two hours after the cerebral procedure completion the patient was claimed by the Comprehensive Stroke Centre to finalize the MT procedure; however, the attempt to recanalize the remaining M2 occlusion was also not successful.
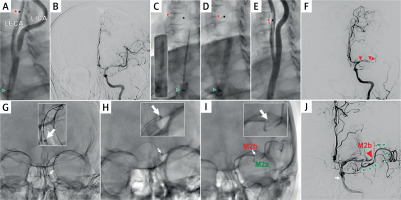
The potential lack of effective protection when using only a mono-balloon catheter positioned in the CCA can be resolved either by using “upfront” a distal filter or by adding a filter to the mono-balloon catheter if there is flow from the ECA to the ICA (Figure 2). This consideration is relevant also in eCAS, as demonstrated in Figure 3 presenting a cerebral embolic complication (middle cerebral artery embolic occlusion) in eCAS in the absence of ECA exclusion. Hence our preference is proximal-protected double balloon (ECA + CCA) system use in both CAS [33, 39] and eCAS [3, 29, 30]. In eCAS the intracranial intervention can usually be performed through the double-balloon catheter (Figure 3). However, it is important to note that ECA exclusion is not relevant to the transcarotid “dynamic” flow reversal systems that require CCA clamping in conjunction with large-bore (lumen radius > 4-fold greater than that in balloon catheter systems) because flow is proportional to the radius to the power 4 (r4). Note the lumen radius of 1.06 mm in MoMa 9F, 1.07 mm in FlowGate 8F, and 1.11 mm in Walrus 8F – vs. 4.5 mm in the TCAR EnRoute dynamic flow reversal system. Thus, in TCAR, the robustness of the reversed flow ensures continuous aspiration from both the ICA and ECA [40, 41].
Finally, the use of an antegrade vs. a retrograde strategy in the management of tandem lesion in the SAFEGUARD-STROKE Study is consistent with the multitude of approaches guided by operator preference and patient anatomy in tandem stroke interventions, as raised by the Buffalo group [31]. One fundamental advantage of the MicroNET-covered stent in eCAS is its sequestration of the atherothrombotic plaque, preventing further plaque-related embolism. We fully agree with our Buffalo colleagues that resolving the carotid pathology before intracranial manipulation is an important contribution to a stepwise approach to prevent new distal embolization in carotid-related strokes and to prevent blind maneuvering into the intracranial circulation during revascularization of tandem lesions [31]. One fundamental disadvantage, however, is the need to cross the carotid stent to reach the intracranial lesion. The latter, as shown in the SAFEGUARD-STROKE study is feasible and can be done safely [30]. Importantly, it may require positioning the guiding catheter distal to the ICA/CCA stent for increased catheter support for the intracranial intervention and – if stentrievers are used – to avoid the risk of entanglement of the stentriever with the stent struts during the stentriever removal. Also, in eCAS, stenting often occurs under limited (for some operators/centers – absent) anticoagulation and with suboptimal antiplatelet protection [9]. Thus optimization of stent embedment with appropriate post-dilatation is crucial to reduce the risk of clot formation [30]. Importantly, for training in acute stroke interventions [42], both the antegrade and retrograde strategy (and their variations) can be pracitised today, including anti-embolic stent use, in a novel human stroke model that can address different clinical scenarios and operator preferences [43].