Introduction
The main reason for dementia is Alzheimer’s disease (AD) that severely affects thinking, memory function, and behavior (Sim, 2014). Approximately 80% of dementia cases are considered to be attributed to AD (Breitner et al., 2009). The precise mechanism for AD induction is not yet well understood yet,; however, the accumulation of amyloid-β peptide in the brain cerebral region is considered to be the main factor of AD pathogenesis (Vaillant-Beuchot et al., 2021).
Apoptosis is one of the primary hallmarks associated with AD incidence. Apoptosis in brain cells such as in the hippocampus affects memory function (Zhang et al., 2020). Two pathways of apoptosis normally occur in mammalian cells under stress, namely intrinsic and extrinsic pathways. One of the enzymes most involved in apoptosis pathways are cysteine proteases such as caspase-3, caspase-8, and caspase-9 (Jiao et al., 2020). Caspase-3 is the main apoptotic enzyme associated with AD (Glushakova et al., 2018). Several molecular tools are used for detecting DNA damage during the apoptosis process such as apoptotic cell death, including TUNEL assay, comet assay, and DNA fragmentation test (Majtnerová and Roušar, 2018).
Other proteins also play an important role in the regulation of apoptosis pathway, such as Bcl-2 family proteins. These proteins are divided into two categories: proapoptotic proteins (such as Bid and Bax) and antiapoptotic proteins (Bcl-2XL and Bcl-2) (Shen et al., 2016). In normal cells, there is a balance between antiapoptotic and proapoptotic members of the Bcl-2 family, which enhances the mitochondrial response to the apoptotic stimuli (Tomita, 2016). Several studies have suggested that the Bax to Bcl-2 ratio is augmented in the hippocampus of AD-induced rats (Ataie et al., 2012; Song et al., 2014).
There is an urgent need to understand the alterations that occur during aging in the brain tissues to develop treatment strategies to mitigate, delay, or prevent neurodegenerative consequences. Molecular pathways associated with the aging process are not well understood, but several processes such as oxidative stress (Zhang et al., 2020) and inflammation (Sochocka et al., 2017) have been recognized as its major reasons. Natural products contain many active compounds that could be used for protection or therapy of several diseases (Saewan and Jimtaisong, 2015). Therefore, there is an increased interest in using natural products against AD because of their high antioxidant capacity, low side effects, and higher safety. Natural polyphenols are expected to be contributive molecules for preventing brain diseases because of their antioxidant activity (Khurana et al., 2013; Rehman et al., 2019).
Among the important flavonoids with high antioxidant activity are quercetin, catechins, kaempferol, and naringenin, which are present in onions, vegetables, and citrus fruits (Manach et al., 2004; Tsao, 2010; Russo et al., 2012; Goufo and Trindade, 2014). Several studies have shown that onion flavonoid intake containing quercetin protects brain tissues against aging through the inhibition of apoptosis that induces brain degeneration (Chae et al., 2012; Wang et al., 2020; Dorrigiv et al., 2021). Moreover, active products present in garlic extracts show protective activity against neurotoxicity (Galal et al., 2019; Hazzaa et al., 2020; Bigham et al., 2021). Furthermore, garlic extracts are known to show activity against aflatoxins (AFs; toxins produced by the fungus Aspergillus flavus ) that induce chronic neurodegeneration in male rats (Alsayyah et al., 2019). Garlic and onions are considered as most important plants with anti-AF and antifungal potential (Abdel-Wahhab and Aly, 2003; Negera and Wash, 2019). Other studies have also been conducted on AD models by using garlic extract and its active ingredients such as S-allyl-L-cysteine (SAC) (Chauhan, 2006; Ray et al. 2011). Garlic extract and its active ingredient SAC mitigated AD by decreasing the amyloid-β peptide content and pathological lesions as well as by increasing the level of antioxidants in the brain tissues of AD models (Borek, 2001). Therefore, the current study aimed to investigate the mitigation effect of onion and garlic root extracts on neurodegenerative diseases in AD models.
Materials and methods
Drugs and chemicals
Aluminum chloride (AlCl3, molecular weight 133.34.) was purchased from Sigma. Trizol was obtained from Invitrogen (Carlsbad, CA, USA). The reverse transcription and PCR kits were obtained from Fermentas (Glen Burnie, MD, USA). SYBR Green Master Mix was purchased from Stratagene (La Jolla, CA, USA). All other chemicals and reagents were of analytical grade and obtained from standard commercial suppliers.
Animals
Ninety male Sprague-Dawley rats aged 15–17 months and weighing 255–310 g (purchased from the Animal House Colony, Giza, Egypt) were maintained on a standard laboratory diet (protein, 16.04%; fat, 3.63%; fiber, 4.1%; and metabolic energy, 0.012 MJ) and water ad libitum at the Animal House Laboratory, National Research Center, Dokki, Giza, Egypt. They were housed in stainless steel wire meshed cages under environmentally controlled conditions. The ambient temperature was 25 ± 2°C, and the light/dark cycle was maintained at 12/12 h. All animals received human care in compliance with guidelines of the Ethical Committee of National Research Centre, Egypt, which follows the recommendations of the National Institutes of Health Guide for Care and Use of Laboratory Animals (Publication No. 85–23, revised 1985). The experiments were approved by the NRC Local Ethical Review Committee (5-12-2020).
Plant materials
Fresh roots of onion (Allium cepa) and garlic (Allium sativum ) were collected from farmers in Wady El-Natron at El-behera governorate, Egypt. The collected roots were dried immediately under sunlight. The onion and garlic samples were authenticated by the Department of Botany, Agriculture and Biology Research Division, National Research Center, Giza, Egypt.
Preparation of root extracts
Methanolic extracts of the onion and garlic roots were prepared by grinding the dried roots (500 g) to a fine powder and mixing with 1000 ml of 80% methanol in a 2000 ml conical flask overnight. Extracts were prepared according to the method of Lacaille-Dubois (Lacaille-Dubois, 2016).
Mycotoxin determination in the extracts
All samples of the collected onion and garlic roots were analyzed for the presence/absence of 3 mycotoxins, including ochratoxin A, AFs (B1, B2, G1, and G2), and zearalenone, by using methods reported previously by Wang et al. (2016) with a minor modification. Briefly, an aliquot of 5.0 g dried roots was mixed with 5 ml of 50 mM citric acid in a 50 ml polypropylene centrifuge tube. Next, 20 ml acetonitrile was added, and the mixture was homogenized with a high-speed blender (Ultra-Turrax T25, IKA, USA) for 3 min. After the addition of 2 g NaCl, the mixture was shaken vigorously for 1 min and centrifuged at 10 000 rpm for 5 min at 10°C. The supernatant was then analyzed for the 3 mycotoxins by UPLC-MS/MS by using the multiple reaction monitoring (MRM) mode. The conditions were set using the BEH Shield RP18 chromatographic column in which the column temperature was 40°C and the flow rate was set at 0.3 ml/min. Mobile phase A was formic acid (0.1%) and ammonium acetate (0.5 mM) aqueous solution. Mobile phase B was formic acid (0.1%) in methanol. The gradient profile was performed with an injection volume of 2 μl.
Antibacterial bioassay
The antibacterial activity test of the collected root extracts was performed using the agar disc diffusion method (Harley and Prescott, 1996). The labeled plates were seeded with the test bacterial organisms (Bacillus cereus, Staphylococcus aureus, Staphylococcus sciuri, Salmonella typhi, Salmonella enterica, Escherichia coli, and Pseudomonas aeruginosa). Sterile filter paper discs of 6 mm diameter were impregnated with various concentrations of the extract (100 and 200 mg/ml) and placed on the seeded plates. The plates were incubated at 37°C for 24 h. After 24 h of incubation, each plate was examined, and the diameters of zones of inhibition were measured in millimeters (mm). The Kirby-Bauer method was used to classify the inhibition zones as follows: inhibitory zones of ≤ 12 mm were regarded as moderately sensitive/ resistant, while those of ≥ 15 mm and above were considered highly sensitive. Plate samples treated with dimethyl sulfoxide (DMSO, 2%) represented negative control, and those treated with tetracycline (500 μg/ml) were used as a positive control.
Antifungal bioautography assay
The antifungal activity of the onion and garlic root extracts was performed according to the method of Wedge et al. (2009). The fungal strains (Aspergillus ochraceus, Aspergillus niger, Fusarium proliferatum, and Penicillium verrucosum ) and root extracts of plants were applied at the dose of 160 μg/spot into a silica plate. Technical fungicide grade standards benomyl, cyprodinil, azoxystrobin, and captan (Chem Service Inc., West Chester, PA, USA) were used as positive controls at 2 mM concentration in 2 μl of 95% ethanol, and sterile potato dextrose agar (PDA, Sigma-Aldrich, St. Louis, MI) was used as a negative control.
Determination of total phenolic content (TPC) in extracts
TPC of the onion and garlic root extracts was assessed using the Folin-Ciocalteu reagent according to the procedure by Makkar (2003). The absorbance of the phenolic content in the extracts was measured at 650 nm using a spectrophotometer (UV mini-1240, UV-vis spectrophotometers, SHIMADZU) against the reagent blank. All measurements were performed in triplicate.
Determination of the total avonoid content (TFC) in extracts
Flavonoid content in onion and garlic root extracts was determined according to the aluminum chloride colorimetric method with a slight modi cation (Zhishen et al., 1999). Briefly, aliquots (4 mg) of onion and garlic root extracts were dissolved in a suitable amount of DMSO, and distilled water was then added to reach a final volume of 2 ml mixture. Approximately 500 μl of the mixture was taken and added to 500 μl distilled water. Next, 300 μl of 5% sodium nitrite was added to the mixture, and the mixture was then allowed to stand for 5 min at room temperature. In the next step, 300 μl of 10% aluminum chloride was added, and the mixture was allowed to stand for 5 min at room temperature. Then, 2 ml of 1M sodium hydroxide was added and incubated for 15 min in dark at room temperature. The absorbance of the sample (pink color developed after incubation) was measured against the reagent blank at 510 nm by using a spectrophotometer. Quercetin was used as a standard in the concentration between 100 and 1000 ppm.
Determination of radical scavenging activity by 2,2-diphenyl-1-picrylhydrazyl (DPPH)
The DPPH radical scavenging activity of onion and garlic root extracts was determined according to the procedure reported by Kumar et al. (2017). The absorbance of DPPH in plant extracts was measured using a spectrophotometer at 517 nm. The capacity to scavenge DPPH radicals was calculated using the following formula:
A0 – negative control absorbance; A1 – sample absorbance.
2, 2 -Azino-bis (3-ethylbenzothiazoline-6-sulphonic acid (ABTS) radical scavenging assay
The ABTS radical scavenging study was performed in plant extracts according to the method of Dhar et al. (2013). The absorbance of ABTS was measured spectrophotometrically at 734 nm. ABTS+ scavenging capacity was calculated as follows:
Abs0 – the absorbance of ethanolic ABTS; Abs1 – the absorbance of ABTS radical with sample extract or standard.
Ferric reducing antioxidant power (FRAP) assay
FRAP assay was performed in plant extracts according to the method of Pulido et al. (2000). Different concentrations (100 and 200 mg/ml) of plant extracts and stock standard solution of 1 mM FeSO4 ∙ 7H2O (positive control) were allowed to react with 2 ml FRAP solution for 30 min at room temperature in dark. All determinations were performed in triplicate. At reaction termination, a blue-colored product (ferrous tripyridyltriazine complex) was formed, indicating the activity of samples against the FRAP reagent. The absorbance of the bluecolored product against the reagent blank was measured at 593 nm by using a spectrophotometer.
Animal experimental design
The rats were randomly assigned to nine groups, with 10 rats in each group. The first group of rats served as the healthy control group. The second group was provided with AlCl3 in drinking water at the dose of 0.3% for 45 days (Erazi et al., 2010); this group served as the AD induced group. After AD induction (45 days of AlCl3 treatment), the AD-induced rats were divided into 7 subgroups: the first to third subgroups of AD-induced rats were administered 1, 2, and 3 mg/kg onion extract through intragastric intubation for 30 days (Alpsoy et al., 2014). The fourth to sixth subgroups of AD-induced rats were administered 1, 2, and 3 mg/kg garlic extract through intragastric intubation for 30 days (Khalil et al., 2008). The seventh subgroup of AD-induced rats was treated with rivastigmine as a reference drug through intragastric intubation at the dose of 0.3 mg/kg b. wt. (Carageorgious et al., 2008) daily for 45 days.
Tissue collection
At the end of the experimental period, the animals were sacrificed under anesthesia, and their brains were rapidly dissected and thoroughly washed with isotonic saline. In each group, half of the brain tissues (5 brains of 5 animals) were fixed immediately after collection in 10% formalin for histopathological examination; the remaining brain tissues (5 brains of 5 animals) were kept at –80°C for further use. Each brain was mid-sagittally divided into two portions. The first portion was stored in liquid nitrogen for molecular analysis, and the second portion was homogenized and centrifuged at 3000 rpm for 10 min under cooling for biochemical analysis.
Histopathological examination of tissue samples
The brain tissue specimens were fixed in 10% formalin for 24 h. Subsequently, the specimens were washed with tap water, prepared, and stained for light microscopy examination as follows: serial dilutions of ethyl alcohol (70, 95, and 100%) were used to hydrate the brain tissues, followed by clearing in xylene. The specimens were then embedded in paraffin wax in a hot air oven at 65°C for 6 h to build tissue blocks, followed by carefully placing of the specimens in cold water (approximately 10°C). The paraffin wax-embedded tissue blocks were then sectioned by using a microtome at the thickness of 5–6 microns. The sections were then collected on glass slides and deparaffinized. Subsequently, the sections were stained for routine histological examination by hematoxylin and eosin staining reagents (Bancroft and Gamble, 2008).
Expression of apoptosis-related genes
Isolation of total RNA
Total RNA was extracted from the brain tissues (50 mg) from the control and treated animals by using the standard TRIzol® reagent extraction method (Invitrogen, Germany). The RNA pellet was dissolved in diethylpyrocarbonate (DEPC)-treated water by passing the solution a few times through a pipette tip. Total RNA was treated with 1 U of RQ1 RNAse-free DNAse (Invitrogen, Germany) to digest DNA residues and then resuspended in DEPC-treated water (100 μl). The purity of total RNA was assessed by monitoring the 260/280 nm ratio (between 1.8 and 2.1). Additionally, the integrity of RNA samples (3 μl) was confirmed with ethidium bromide staining (5 μl/100 ml: 2% agarose) analysis of 28S and 18S bands by formaldehyde-containing agarose gel electrophoresis. RNA aliquots were used immediately for reverse transcription (RT) (Salem et al., 2018).
RT reaction
Five micrograms of the complete total RNA isolated from each animal sample was reverse transcribed into cDNA in a total volume of 20 μl by using the RevertAidTM First Strand cDNA Synthesis Kit (MBI Fermentas, Germany) with 50 μM oligo-dT primer. The RT reaction was performed at 25°C for 10 min, followed by 1 h at 42°C, and the reaction was stopped by heating for 5 min at 99°C. The reaction tubes containing RT preparations were then flash-cooled in an ice chamber until their use for DNA amplification through quantitative real time-polymerase chain reaction (qRT-PCR) (Khalil et al., 2018).
qRT-PCR
PCR reactions were set up in 25 μl reaction mixtures containing 12.5 μl of 1 × SYBR® Premix Ex TaqTM (TaKaRa Biotech Co. Ltd., Germany), 0.5 μl of 0.2 μM sense primers, 0.5 μl of 0.2 μM antisense primer, 6.5 μl of distilled water, and 5 μl of cDNA template. At the end of each qRT-PCR, a melting curve analysis was performed at 95°C to check the quality of the primers used for the Bax, Bcl2, and caspase 3 genes. The primers sequences of the Bax and caspase-3 genes were designed using Primer3 software (Primer3 Input, version 0.4.0), while the primer sequences of Bcl2 and β-actin were selected according to Khalil and Booles (2011) – Table 1. Each experiment included a distilled water control. The quantitative values of RT-PCR (qRT-PCR) of apoptosisrelated genes were normalized on the basis of ß-actin expression level (Khalil and Booles, 2011). The relative quantification of the target to the reference was determined using the 2-∆∆CT method.
Table 1
Primer sequences used for qPCR
Gene | Primer sequence (5′–3′) | References/NCBI |
---|---|---|
Bax | F: CGA GCT GAT CAG AAC CAT CA | NM-017059.2 |
R: CTC AGC CCA TCT TCT TCC AG | ||
Bcl2 | F: CTC AGT CAT CCA CAG GGC GA | Khalil and Booles (2011) |
R:AGA GGG GCT ACG AGT GGG AT | ||
Caspase 3 | F: GGA CCT GTG GAC CTG AAA AA | NM-012922.2 |
R: GCA TGC CAT ATC ATC GTC AG | ||
β-actin | F: CAC GTG GGC CGC TCT AGG CAC CAA | Khalil and Booles (2011) |
R: CTC TTT GAT GTC ACG CAC GAT TTC |
Comet assay
Comet assay was performed according to the protocol by Blasiak et al. (2004), with minor modifications. The brain tissues of the rats (500 mg) were homogenized in a lysis buffer. Next, 50–100 μl of the homogenized tissues of each treatment were mixed with low-meltingpoint agarose (ratio of 1 : 10 v/v) and then pipetted to precoated slides with normal-melting-point agarose. The slides were kept flat at 4°C for 30 min in dark. The third layer of low-melting-point agarose was then pipetted on slides and left to solidify for 30 min at 4°C. The slides were then transferred to a pre-chilled lysis solution and kept for 60 min at 4°C. Subsequently, the slides were immersed in a freshly prepared alkaline (pH > 13) unwinding solution (200 mM NaOH, 1 mM EDTA) at room temperature in dark for 60 min. The slides were then subjected to an electrophoresis run at 0.8 V/cm, 300 mAmps at 4°C for 30 min. The slides were subsequently rinsed in a neutralizing solution (0.4 mM Tris, pH 7.5) followed by immersion in 70% ethanol and then air-dried. These slides were then stained with an aqueous solution of ethidium bromide dye (0.02 mg/ml) and visualized by a Zeiss epifluorescence microscope (510–560 nm, barrier filter 590 nm) with a magnification of × 400 to determine DNA damage in 100 cells per animal.
Determination of ROS formation
Intracellular ROS generation was measured in brain tissues by a flow cytometer with an oxidation-sensitive dichlorofluorescein diacetate (DCFH-DA) fluorescent probe. Single-cell suspensions were prepared by pipetting homogenized tissues for 1–2 min to obtain suspension cells (Khalil and Abdu, 2013). DCFH-DA is a nonfluorescent compound that is freely taken up into brain cells. DCFH is oxidized to fluorescent dichlorofluorescein (DCF) through the action of cellular oxidants. The suspension was loaded with the DCFH-DA solution to a final concentration of 50 μM, and the solution was then incubated for 30 min at 37°C. The samples were then centrifuged at 1000 rpm for 5 min (4°C), and the cells were resuspended in phosphate-buffered saline (PBS, pH 7.2–7.4). The fluorescence was detected by a flow cytometer (with excitation at 488 nm and emission at 525 nm). For each treatment, 1 × 105 cells were counted, and the experiment was performed in triplicate.
Statistical analysis
All data were analyzed using the General Linear Model (GLM) procedure of Statistical Analysis System (SAS, 1982) followed by the Scheffé test to assess significant differences between the groups. The values are expressed as mean ± SEM. All significance values were based on the probability of P < 0.05.
Results
Mycotoxin determination in the root plant extracts
The test results showed that the three mycotoxins (ochratoxin A, AFs (B1, B2, G1, and G2), and zearalenone) were not detected in both onion and garlic fresh root extracts.
Antifungal and antibacterial activities of the root plant extracts
Tables 2 and 3 show the antibacterial and antifungal activities of the onion and garlic root extracts. Three gram-positive foodborne pathogenic bacteria (Bacillus cereus EMCC 1080, Staphylococcus aureus ATCC 13565, and Staphylococcus sciuri 2–6) and four gram-negative bacteria (Salmonella typhi ATCC 25566, Salmonella enterica SA19992307, Escherichia coli 0157 H7 ATCC 51659, and Pseudomonas aeruginosa NRRL B-272) were used to test the antibacterial activities of the onion and garlic root extracts (Table 2). The results showed that the onion root extracts exhibited the highest mean values of bacterial growth inhibition (11.8 ± 0.76 and 14.8 ± 1.04 at 100 and 200 mg/ml, respectively) against E. coli. The second and third highest mean growth inhibition values of the onion root extracts were observed for S. aureus (10.7 ± 1.52 and 12.0 ± 1.32) and P. aeruginosa (9.5 ± 0.86 and 13.2 ± 1.25) at 100 and 200 mg/ml concentrations, respectively. The highest mean growth inhibition value of the garlic root extracts was observed for E. coli (10.2 ± 0.76 and 11.2 ± 0.76). The second highest mean growth inhibition value of the garlic root extracts was noted for S. aureus (8.7± 0.50 and 11.2 ± 0.76) at 100 and 200 mg/ml concentrations, respectively.
Table 2
Antibacterial activity of methanolic onion and garlic root extracts against several pathogenic bacteria
Bacteria | Growth inhibition (mean ± SEM) | |||||
---|---|---|---|---|---|---|
negative control | positive control | onion | garlic | |||
100 mg/ml | 200 mg/ml | 100 mg/ml | 200 mg/ml | |||
B. cereus | 0 | 16.6 ± 0.58 b | 11.5 ± 0.86 a | 12.7 ± 1.25 ab | 11.5 ± 0.50 a | 13.2 ± 0.76 a |
Staph. aureus | 0 | 18.0 ± 1.04 ab | 10.7 ± 1.52 a | 12.0 ± 1.32 ab | 8.5 ± 0.50 ab | 11.2 ± 0.76 ab |
Staph. sciuri | 0 | 17.7 ± 0.86 ab | 8.5 ± 0.50 ab | 12.2 ± 1.04 ab | 7.8 ± 0.28 b | 9.8 ± 1.04 b |
E. coli | 0 | 18.8 ± 1.14 ab | 11.8 ± 0.76 a | 14.8 ± 1.04 a | 10.2 ± 0.76 a | 11.2 ± 0.76 ab |
S. typhi | 0 | 15.5 ± 0.50 b | 8.2 ± 0.76 ab | 11.2 ± 0.76 ab | 8.8 ± 1.61 ab | 10.5 ± 0.86 ab |
S. enterica | 0 | 16.0 ± 1.00 ab | 7.8 ± 0.76 b | 9.2 ± 9.20 b | 8.5 ± 0.86 ab | 9.3 ± 1.25 b |
P. aeruginosa | 0 | 19.2 ± 0.58 a | 9.5 ± 0.86 ab | 13.2 ± 1.25 a | 8.7 ± 0.86 ab | 11.3 ± 1.04 ab |
Table 3
Antifungal activity of the methanolic onion and garlic root extracts against several pathogenic fungi
Fungal | Growth inhibition (mean ± SEM) | |||||
---|---|---|---|---|---|---|
negative control | positive control | onion | garlic | |||
100 mg/ml | 200 mg/ml | 100 mg/ml | 200 mg/ml | |||
A. ochraceus | 0 | 21.0 ± 0.50 a | 7.3 ± 0.28 | 8.5 ± 0.50 | 8.2 ± 1.04 | 9.5 ± 1.00 |
A. niger | 0 | 22.8 ± 2.55 a | 8.5 ± 0.50 | 9.3 ± 0.58 | 7.5 ± 0.50 | 8.0 ± 0.50 |
F. proleferatum | 0 | 17.5 ± 0.86 b | 7.8 ± 0.28 | 9.0 ± 0.50 | 7.7 ± 0.76 | 9.0 ± 0.50 |
P. verrucosum | 0 | 13.8 ± 01.75 c | 7.8 ± 0.14 | 8.5 ± 0.50 | 7.2 ± 0.36 | 7.7 ± 0.28 |
Different strains of fungi (Aspergillus ochraceus ITAL 14, Aspergillus niger IMI288550, Fusarium proliferatum MPVP 328, and Penicillium verrucosum BFE 500) were used to determine the antifungal activities of the onion and garlic root extracts. The results showed no significant differences (P > 0.05) in the mean values of growth inhibition between the onion and garlic root extracts against all the tested strains of fungi (A. ochraceus, A. niger, F. proliferatum, and P. verrucosum).
TPC and TFC in the methanolic onion and garlic root extracts
TPC and TFC were determined in the methanolic onion and garlic root extracts. The results showed that garlic root extract had higher levels (P < 0.05) of TPC (542.2 ± 2.95) and TFC (112.0 ± 2.00) than the onion root extracts (TPC: 406.3 ± 2.58; TFC: 42.7 ± 1.15).
DPPH and ABTS radical scavenging activities and FRAP antioxidant levels
The levels of DPPH and ABTS free radical scavenging activities and the antioxidant levels based on FRAP assay were determined for the onion and garlic root extracts. The DPPH scavenging activity levels were relatively similar for both plant extracts (garlic: 11.4 ± 0.05; onion: 10.2 ± 0.34). However, the ABTS scavenging activity levels of the garlic root extract were significantly (P < 0.05) higher (98.1 ± 1.04) than those of the onion root extract (77.9 ± 2.58). Moreover, the garlic root extract showed considerably (P < 0.05) higher antioxidant levels (99.3 ± 2.01) in the FRAP assay than the onion root extract (76.2 ± 1.08).
Histopathological findings
The brain sections of the control negative group revealed normal brain tissues without any lesions (Fig. 1A). However, the pyramidal cells of the hippocampus of AD animals exhibited degeneration and pyknosis with neurofibrillary tangles (arrow, Fig. 1B). Treatment of AD-induced animals with a low dose (1 mg/kg) of the onion root extract led to induction of necrobiosis of astrocytes (arrow) and focal neurofibrillary tangles of large neurons (Fig. 1C). AD-induced animals treated with a medium dose (2 mg/kg) of the onion root extract had necrobiosis of neurons and neurofibrillary tangles that were observed in some degenerated cells in addition to deposits of eosinophilic materials (Fig. 1D). Importantly, the brain sections of AD-induced rats treated with a high dose (3 mg/kg) of the onion root extract showed relatively normal pyramidal and neuron cells of the hippocampus (Fig. 1E).
Fig. 1
Representative photomicrographs of brain sections stained with hematoxylin-eosin stain: A) brain section of the control negative group (magnification 400 ×); B) brain sections of AD-induced animals (400 ×); C) brain sections of AD-induced rats treated with a low dose of the onion root extract (400×); D) brain sections of AD-induced rats treated with a medium dose of the onion root extract (200 ×); E) brain sections of AD-induced rats treated with a high dose of the onion root extract (100 ×); F) brain sections of AD-induced rats treated with a low dose of garlic root extract (400 ×); G) brain sections of AD-induced rats treated with a medium dose of the garlic root extract (100×); H) brain sections of AD-induced rats treated with a high dose of the garlic root extract (200 ×); I) cerebellum sections of AD-induced rats treated with the reference drug rivastigmine (400 ×)
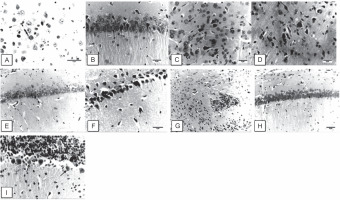
On the other hand, the brain sections of AD-induced rats treated with a low dose (1 mg/kg) of garlic root extract had neuronal degeneration and pyknosis in the hippocampal cells. Neurofibrillary tangles of the hippocampal pyramidal neurons were also detected (Fig. 1F). Representative photomicrographs of the brain sections of AD-induced rats treated with a medium dose (2 mg/kg) of the garlic root extract revealed focal cerebral necrosis with infiltration of glial cells (star) (Fig. 1G). Brain sections of AD-induced rats treated with a high dose (3 mg/kg) of the garlic root extract showed slight degeneration of the hippocampal neuron cells (Fig. 1H). The representative photomicrographs of the cerebellum sections of AD-induced rats treated with the reference drug rivastigmine observed Purkinje cells with necrobiosis (arrows, Fig. 1I).
Alteration in the expression of apoptosis-related genes
Expression patterns of the apoptotic genes Bax, Bcl2, and caspase-3 in the brain tissues of AD-induced rats treated with different concentrations of the onion and garlic root extracts are summarized in Figures 2–4.
Fig. 2
Alterations in the expression levels of the Bax gene in brain tissues collected from AD-induced rats treated with low (L – 1 mg/kg), medium (M – 2 mg/kg), and high (H – 3 mg/kg) doses of the onion and garlic root extracts; data are presented as mean ± SEM; a, b, c, d – mean values within the tissue with different superscript letters were significantly different (P < 0.05)
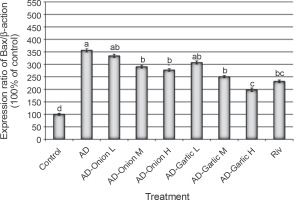
Fig. 3
Alterations in the expression levels of the Bcl2 gene in brain tissues collected from AD-induced rats treated with low (L – 1 mg/kg), medium (M – 2 mg/kg), and high (H – 3 mg/kg) doses of the onion and garlic root extracts; data are presented as mean ± SEM; a, b, c, d – mean values within the tissue with different superscript letters were significantly different (P < 0.05)
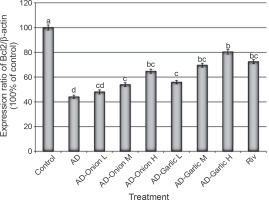
Fig. 4
Alterations in the expression levels of the caspase-3 gene in brain tissues collected from AD-induced rats treated with low (L – 1 mg/kg), medium (M – 2 mg/kg), and high (H – 3 mg/kg) doses of the onion and garlic root extracts; data are presented as mean ± SEM; a, b, c, d, e, f – mean values within the tissue with different superscript letters were significantly different (P < 0.05)
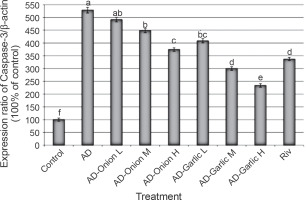
The expression levels of the Bax (356.6%) and caspase-3 (528.6%) genes were significantly higher, but those of the Bcl2 (44.1%) gene was lower in the brain tissues of AD-induced rats as compared that (100%) in the control group (P < 0.01). However, treatment of AD-induced rats with medium (2 mg/kg) and high (3 mg/kg) doses of the onion root extract decreased the expression levels of Bax (290.6 and 277.4%, respectively) and caspase-3 mRNAs (448.6 and 374.3%, respectively) and increased the expression levels of the Bcl2 gene (53.9 and 64.7%, respectively) as compared to that in AD-induced rats. The reduction in the expression levels of Bax and caspase-3 mRNAs and the increase in the Bcl2 gene expression in AD-induced rats treated with a low dose (1 mg/kg) of the onion root extract were nonsignificant (P > 0.05) as compared to those in AD-induced rats (Fig. 2–4).
Treatment of AD-induced rats with low, medium, and high doses (1, 2, and 3 mg/kg) of the garlic root extract significantly decreased the expression levels of Bax (307.5, 250.9, and 198.1%, respectively) and caspase-3 (408.6, 300, and 234.3%, respectively) and increased the levels of Bcl2 mRNA (55.9, 69.6, and 80.4%, respectively) in their brain tissues as compared to that in the brains of AD-induced rats. Moreover, the lowest expression levels of Bax and caspase-3 genes and the highest expression level of the Bcl2 gene were observed in AD-induced rats treated with a high dose of the garlic root extract. Treatment of AD-induced rats with rivastigmine also significantly decreased the expression levels of the Bax (232.1%) and caspase-3 (337.1%) genes and increased the levels of the Bcl2 (72.5%) gene as compared to those in untreated AD-induced rats (Fig. 2–4).
Effect of the root extracts on DNA damage as assessed by comet assay
The damage rate of DNA in the brain tissues of AD-induced rats treated with the onion and garlic root extracts is summarized in Table 4. The rates of DNA damage in the brain tissues of AD-induced rats increased (23.4 ± 0.51) significantly (P < 0.01) as compared to that in the control rats (6.8 ± 0.25). Treatment of AD-induced rats with medium and high doses (2 and 3 mg/kg) of the onion root extract significantly decreased DNA damage (17.8 ± 0.37 and 14.6 ± 0.68, respectively) in their brain tissues as compared to that in the brains of AD-induced rats (23.4 ± 0.51). AD-induced rats treated with a low dose (1 mg/kg) of the onion root extract showed a slight decrease in DNA damage (21.2 ± 0.58) as compared to that in untreated AD-induced rats (23.4 ± 0.51).
Table 4
Rate of DNA damage in the brain tissues of AD-induced rats treated with low (L), medium (M), and high (H) doses of onion and garlic root extracts by using comet assay
Treatment | Number of cells * | Class¥ of comet | DNA damaged cells (mean ± SEM) | ||||
---|---|---|---|---|---|---|---|
analyzed | total comets | 0 | 1 | 2 | 3 | ||
Control | 500 | 34 | 466 | 28 | 6 | 0 | 6.8 ± 0.25 e |
AD | 500 | 117 | 383 | 34 | 46 | 37 | 23.4 ± 0.51 a |
AD-Onion L | 500 | 106 | 394 | 38 | 43 | 25 | 21.2 ± 0.58 a |
AD-Onion M | 500 | 89 | 411 | 35 | 28 | 26 | 17.8 ± 0.37 b |
AD-Onion H | 500 | 73 | 427 | 42 | 22 | 9 | 14.6 ± 0.68 c |
AD-Garlic L | 500 | 87 | 413 | 36 | 27 | 24 | 17.4 ± 0.60 b |
AD-Garlic M | 500 | 66 | 434 | 32 | 21 | 13 | 13.2 ± 0.58 c |
AD-Garlic H | 500 | 54 | 446 | 28 | 20 | 6 | 10.8 ± 0.74 d |
Riv | 500 | 81 | 419 | 29 | 30 | 22 | 16.2 ± 0.58 bc |
¥ Class: 0 – no tail, 1 – tail length < diameter of nucleus, 2 – tail length between 1× and 2× the diameter of nucleus, and 3 – tail length > 2× the diameter of nucleus;
Treatment of AD-induced rats with low, medium, and high doses (1, 2, and 3 mg/kg) of the garlic root extract considerably decreased DNA damage (17.4 ± 0.60, 13.2± 0.58, and 10.8 ± 0.74, respectively) in their brain tissues as compared to that in untreated AD-induced rats (23.4 ± 0.51). The lowest rate of DNA damage (10.8 ± 0.74) found in the group of AD-induced rats treated with a high dose (3 mg/kg) of the garlic root extract, which was relatively close to that observed in control rats (6.8 ± 0.25).
Effects of the onion and garlic root extracts on ROS generation in AD-induced rats
Figure 5 shows the effects of the onion and garlic root extracts on the intracellular ROS fluorescence intensity (%). The mean fluorescence intensity (%) of intracellular ROS in AD-induced rats was significantly higher (274%) than that in the control group (41%). Treatment of AD-induced rats with different doses of the onion and garlic root extracts significantly decreased the mean fluorescence intensity (%) of intracellular ROS as compared with that in untreated AD-induced rats. The mean fluorescence intensity (%) of intracellular ROS in AD-induced rats treated with medium and high doses (2 and 3 mg/kg) of the onion root extract was reduced considerably (165 and 142%) as compared to that in AD-induced rats (274%). Moreover, the mean fluorescence intensity (%) of intracellular ROS in AD-induced rats treated with low, medium, and high doses (1, 2, and 3 mg/kg) of the garlic root extract decreased significantly (168, 124 and 77%, respectively) as compared to that in untreated AD-induced rats (274%). The results showed that lowest intracellular ROS fluorescence intensities were observed in the group of AD-induced rats treated with a high dose of the garlic root extract (77%) and rivastigmine (86%) – Figure 5.
Fig. 5
Alterations in intracellular ROS levels in the brain tissues of AD-induced rats treated with low (L – 1 mg/kg), medium (M – 2 mg/kg), and high (H – 3 mg/kg) doses of onion and garlic root extracts; data are presented as mean ± SEM; a, b, c, d, e, f – within each column, mean values with different superscript letters are significantly different (P < 0.05)
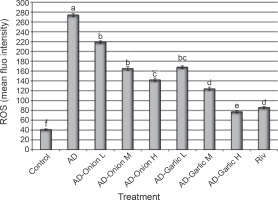
Discussion
AD is one of the chronic diseases with increasing incidence annually worldwide. Neuronal degeneration in the brain tissues occur due to several reasons. AF is a fungal toxin produced by the fungus Aspergillus that is widely found in improperly stored feed and food (Wei et al., 2018). Recently, Huang et al. (2020) found that aflatoxin-B1 (AFB1) causes brain damage mediated by ROS generation and DNA damage. Therefore, in the present study, we focused on plant extracts such as onion and garlic root extracts with antifungal activities as promising natural products against AD. Alsayyah et al. (2019) reported that garlic extracts showed protection against AF-induced chronic neurodegeneration in male rats. In the current study, the capability of the onion and garlic root extracts to exhibit antibacterial and antifungal activities revealed that these extracts showed high growth inhibition against different bacterial and fungal strains. Many studies have investigated the use of natural compounds extracted from several medicinal plants (ginger, Trachyspermum ammi (L.) Sprague, and Cymbopogon citratus) against fungal growth and production of AF (Velazhahan et al., 2010; Janardhan et al., 2016; Vipin et al., 2017). Moreover, garlic extracts were shown to prevent AF production (Abdel-Wahhab and Aly, 2003; Negera and Wash, 2019). Onion extracts also exhibited antifungal and anti-AF activities (Abdel-Wahhab and Aly, 2003). Thus, previous studies have confirmed that several plants such as ginger (Mohd Sahardi and Makpol, 2019), Trachyspermum ammi (Bairwa et al., 2012), citrus fruits (Elumalai and Lakshmi (2016), and onion and garlic (Luo et al., 2021) can protect neural cells against toxicity.
Apoptosis is one of the main hallmarks of AD (Zhang et al., 2020). The enzymes belonging to the cysteine protease family are among the important enzymes that play a vital role in the mechanism of apoptosis (Crowley and Waterhouse N.J., 2016). One of these enzymes is caspase-3 which is necessary for the activation of other apoptotic enzymes such as caspase-8 and caspase-9 (Wan et al., 2019). Because of its vital role in AD and to understand the mechanism of AD, caspase-3 is one of the most studied apoptotic enzymes, because it is associated with neurotoxicity in the brain tissues (Szychowski et al., 2019). The findings of the current study indicated that the expression levels of the apoptosis-related genes such as the caspase-3 and Bax genes increased significantly in the brain tissues of AD-induced rats as compared to those in the control group.
The current study found that the treatment of AD-induced rats with several doses of onion and garlic root extracts decreased the expression levels of the Bax (Fig. 2) and caspase-3 (Fig. 4) genes as compared to those in untreated AD-induced rats. The protective effect of these extracts might be attributed to its content of flavonoids that act as important antioxidants) Golubkina et al., 2020).
The present results also indicated that the protective effect of the garlic root extract against apoptosis was more clearer than that of the onion root extract. The expression levels of the caspase-3 and Bax genes in the brain tissues of AD-induced rats treated with the garlic root extract decreased more than that in AD-induced rats treated with the onion root extract. Kim et al. (2018) conducted a comparative study between the antioxidant activities and bioactive organosulfur compounds in garlic and onion and found that the garlic extract had a significantly high level of γ-glutamyl peptides as compared to the onion extract. γ-Glutamyl peptides are associated with the glutathione (GSH) cycle and metabolic enzymes such as γ-glutamyl cysteine synthetase and γ-glutamyl transpeptidase (Yang et al., 2019). The present study also found that the garlic root extract had much higher levels of TFC and TPC than the onion root extract. Thus, the higher protective role of the garlic root extract than that of the onion root extract could be attributed to the high percentage of antioxidant compounds in the former.
The protective role of onion against neurodegeneration can be attributed to its active ingredients such as flavonoids. Among the main active flavonoids are quercetin, catechins, kaempferol, and naringenin, which are present in onions and some other vegetables and citrus fruits (Manach et al., 2004; Tsao, 2010; Russo et al., 2012; Goufo and Trindade, 2014). Several studies have been conducted on the effect of quercetin as an active flavonoid in onion against neurodegenerative diseases (Schmitt-Schillig et al., 2005; Tsao, 2010; Russo et al., 2012; Goufo and Trindade, 2014). The researchers found that onion flavonoid intake (including quercetin) prevented brain aging through the inhibition of several biochemical processes that affect brain diseases. As confirmed in numerous studies, the mechanism of action of quercetin extracted from onion is through the promotion of the antioxidant activity and prevention of intracellular ROS generation (Russo et al., 2012, Lu et al., 2017). The current study also confirmed the presence of flavonoids in the onion root extract, thus indicating its potential in inhibiting ROS generation in the brain tissues of the AD rat model.
Several studies have indicated that quercetin enhances protective effects against several pathological diseases such as cancer and diabetes (Kobori et al., 2009; Russo et al., 2012) through improvement in antioxidant capacity and regulation of the expression patterns of Nrf2 and PON2 (Zaplatic et al., 2019; Costa et al., 2016). Quercetin content was determined in the brain tissues of rats supplemented orally with quercetin, and it was found to play an effective role in alleviating neurodegenerative disorders (De Boer et al., 2005). The present study found that treatment of AD-induced rats with garlic root extracts decreased histopathological brain lesions (Fig. 1), decreased the expression levels of the apoptotic genes (Figs. 2–4), promoted nucleic acid repair through decrease in DNA damage rate (Table 4), and inhibited intracellular ROS generation in brain tissues (Fig. 5). This effect could be attributed to our findings, thus showing that the garlic root extract had higher free radical ABTS scavenging activity and antioxidant FRAP levels than that of the onion root extract. These results are in line with other studies conducted on AD rat models and treated with garlic extracts (Pavitt et al., 1998; Costa et al., 2016). These studies indicated that the protective effect of garlic extracts can be attributed to its active ingredient, namely SAC. The garlic extract and its active ingredient SAC can alleviate brain aging by decreasing the content of amyloid-β peptide and reducing pathological lesions (Holcik and Sonenberg, 2005). Borek (2001) also suggested that the mechanism of action of garlic extract and/or SAC is attributed to increase in the level of antioxidants in the brain tissues of AD models. Hermawati et al. (2015) suggested the role of the active ingredient SAC in garlic as it promotes an increase in the level of antioxidant enzymes such as glutathione peroxidase (GPx), glutathione reductase (GR), and superoxide dismutase (SOD) mediated by upregulation of the Nrf2 factor in the hippocampus of male rats.
Kang et al. (2016) used garlic extract to mitigate oxidative stress and reported that the presence of saponin in garlic extract suppressed intracellular ROS generation and consequently decreased DNA damage in mouse brain cells. The mechanism of action of garlic saponin against oxidative stress was attributed to the enhancement of the antioxidant capacity through an increase in the level of heme oxygenase-1 (HO-1), which is an important antioxidant enzyme against cellular oxidative damage (Kang et al., 2016). Park et al. (2009) reported that the protective effect of garlic against cellular oxidative stress-induced genotoxicity was because of its capacity to improve the antioxidant activity. Abdella and Gad (2008) found that the antigenotoxic effect of garlic extract could be due to the presence of sulfhydryl groups in its extract materials, which prevented the generation of free radicals and thus suppressed DNA damage. The higher protective role of the garlic root extract against AD than that of the onion root extract could be attributed to the high levels of γ-glutamyl peptides (Yang et al., 2019), high TFC and TPC, high free radical scavenging of ABTS and antioxidant FRAP levels, and high levels of SAC (Ray et al. 2011) and saponin (Kang et al. 2016) in the garlic root extract as compared to that in the onion root extract.
Conclusions
Supplementation of AD-induced rats with onion and garlic root extracts mitigated the toxic symptoms observed in these rats in the form of histopathological lesions, alterations in the expression levels of the apoptotic genes, DNA damage, and intracellular ROS generation in brain tissues. The protective role of onion root extract against AD might result from its content of flavonoid compounds that improve the antioxidant capacity and regulation of the gene expression patterns. Further studies are required on the garlic and onion extract fraction for better understanding of the mechanism of the specific bioactive compounds present in these extracts against AD.