Introduction
Colorectal cancer (CRC) stands as the second most prevalent cancer in women and the third in males, ranking as the second-leading cause of cancer-related mortality worldwide [1]. Malignant tumours share common traits, including persistent proliferation, angiogenesis, and the evasion of apoptosis by tumour cells [2].
Angiogenesis, a fundamental process in tumour development, involves the formation of new blood vessels that supply oxygen and nutrients to the growing tumour [2]. One of the key players in angiogenesis is vascular endothelial growth factor (VEGF), which is implicated in various aspects of cancer progression. Vascular endothelial growth factor signalling pathways are involved in immunosuppression, tumour metabolism, angiogenesis, cell proliferation, apoptosis, and drug resistance [2–4].
Vascular endothelial growth factor activates multiple kinase signalling pathways, including PI3K/AKT, protein kinase C, extracellular signal-regulated kinase (ERK), nuclear factor kappa B (NF-κB), and activated mitogen protein kinase (AMPK), which collectively facilitate the proliferation, migration, and survival of endothelial cells during angiogenesis [5–6].
Activated mitogen protein kinase, a master regulator of cellular energy, is a heterotrimeric kinase complex composed of a catalytic α-subunit and two regulatory subunits [6]. Under conditions of low energy, AMPK phosphorylates specific enzymes and growth control nodes to enhance ATP synthesis and reduce ATP consumption. The identification of the relationship between AMPK and cancer was driven by the tumour-suppressive actions of the liver kinase B1 enzyme, which phosphorylates AMPK and inhibits mTORC1 [7].
Regorafenib, an inhibitor of VEGF signalling, received approval from the US Food and Drug Administration in September 2012 [8] as a salvage therapy for CRC patients who had previously undergone chemotherapy with or without anti-VEGF or anti-epidermal growth factor receptor (EGFR) therapy. However, regorafenib resistance is on the rise among patients, posing a significant challenge to effective treatment [9]. To overcome this, regorafenib is often combined with other anticancer medications to enhance the efficacy of CRC therapy [10].
Studies have highlighted the synergistic anticancer effects that natural medicine monomers can exhibit when combined with chemotherapeutic drugs. For example, the influence of sigmasterol on sorafenib-treated human breast cancer cell lines [11] and the potential of rhamnazin to enhance the antitumour effects of sorafenib-treated human hepatocellular carcinoma (HCC) cell lines [12].
All-trans retinoic acid (ATRA), a primary metabolite of vitamin A, has shown promise as a chemotherapeutic and chemopreventive agent in the treatment and prevention of cancer [13, 14]. It has demonstrated potential in various cancer types, such as breast cancer, glioma, and head and neck cancer [15]. Notably, ATRA administration can activate the ERK signalling pathway through a transcription-independent manner, involving RAR and PI3K, thereby promoting lung cancer cell proliferation, survival, and migration [16]. However, the impact of ATRA treatment varies across different cancer types, such as lung cancer, potentially due to the dual effects of activated ERK on cancer growth and the genetic diversity observed in various cancer types [17].
Material and methods
Chemicals and therapeutic agents
Regorafenib (BAY 73-4506) was procured from Selleck-Chem. All-trans retinoic acid, foetal bovine serum (FBS), and dimethyl sulfoxide (DMSO) were obtained from Sigma-Aldrich. 3- (4-, 5-Dimethylthiazolyl-2)-2, 5-diphenyltetrazolium bromide (MTT) was purchased from Serva. Dulbecco’s modified Eagle’s medium (DMEM), trypsin, buffered saline, and a penicillin/streptomycin mixture were procured from Lonza.
Cell lines and culture conditions
Two human CRC cell lines, Caco-2 and HCT-116, were sourced from the American Type Culture Collection following the instructions of the ethical committee of the faculty of pharmacy at Damanhur University (ref No. 220PB16). Cells were cultured in high-glucose DMEM supplemented with 10% FBS and 1% penicillin/streptomycin at 37°C in the presence of 5% CO2 and 95% humidified air [18]. Cells were passaged around 5–6 times after reaching 90% confluence. Regorafenib and ATRA were dissolved in DMSO (1%), and then DMEM was added to obtain the required concentrations. Dulbecco’s modified Eagle’s medium with a similar quantity of DMSO used in treated groups (1%) was used as a control.
All experiments were conducted as 3 separate experiments, each of which was performed in triplicate.
Cell viability assay
Caco-2 and HCT-116 cells were tested in vitro for sensitivity to regorafenib, ATRA, or a combination of the 2 drugs using a modified MTT assay [19, 20]. Exponentially growing cells were seeded in 96-well plates with a seeding density of 5 × 103/well, and the plates were incubated overnight at 37°C [21]. Twenty-four hours later, old media were discarded, followed by the addition of 100 µl of media containing regorafenib concentrations (1, 3, 5, 7, and 8) µg/ml for HCT-116 and Caco-2 cells, along with ATRA concentrations of (80, 90, 100, 110, and 150) µg/ml for both cell lines, to different wells. Then, the plates were maintained in the incubator at 37°C for a further 48 hours. Cell viability was measured for each individual drug and combination after 48 h in both Caco-2 and HCT-116 cell lines.
To measure cell viability, 5 mg/ml of MTT was added to each well of the plates for 4 hours. Subsequently, the media were replaced with 100 µl of DMSO and gently agitated in the dark for 20 minutes to dissolve the formed formazon crystals [22]. The absorbance was measured at λ max 570 nm (A570 nm) using an automated enzyme-linked immunosorbent assay (ELISA) microplate reader (Bio-Rad).
All experiments were conducted in triplicate and repeated in 3 independent experiments. Cell viability was represented as a percentage relative to the control. The IC50 values, representing the concentration required to induce cell death in 50% of the cells, were determined using Graph Pad Prism 7.
Experimental cell design
Cells from each individual cell line were initially cultured in 15 T-75 flasks at a density of 2 × 10^5 cells per flask. After an overnight incubation, the flasks were categorized into 4 groups, each with a unique experimental condition. The first group served as the control and was treated with a 1% DMSO solution. In contrast, the second group underwent treatment with ATRA. The third group received treatment with regorafenib, with concentrations tailored to their respective IC50 levels. Lastly, the fourth group was subjected to a combination treatment involving ATRA and regorafenib at sub-IC50 doses (100 µM ATRA + 5 µM regorafenib). These cells were allowed to incubate for a duration of 48 hours. After this incubation period, the cells were carefully harvested and then subdivided into various aliquots, all of which were meticulously preserved at a frigid –80°C. These aliquots were kept in this state until they were utilized in the subsequent experiments and analyses.
Apoptosis assay (annexin-v-FITC/propidium iodide assay)
To assess apoptosis induction by regorafenib, ATRA, or their combination, an annexin-V assay via flow cytometry was employed. Cells were initially seeded at a density of 3 × 105 cells in a 6-well plate and allowed to adhere for 24 hours at 37°C [23]. Following adherence, the cells were treated with regorafenib, ATRA, or a combination of both, as per the experimental design, for 48 hours. Subsequently, the cells were trypsinized, collected through centrifugation at 2000 rpm, and stained with annexin V-FITC and propidium iodide according to the manufacturer’s protocol. Analysis of apoptotic cells involved gating 20,000 cells via flow cytometry and recording FITC and PI fluorescence signals. The data obtained was then analysed using CytExpert Software.
Cell lysates preparation
Cell lysates were prepared using RIPA lysis buffer. For each cell line, Caco-2 and HCT-116, 0.5 ml of RIPA lysis buffer was added to the cell pellets. The mixtures were vortexed and subsequently incubated on ice for 30 minutes. Afterward, the samples were centrifuged at 14,000 g for 10 minutes to remove cellular debris, and the resulting lysates were stored at –20°C until further analysis [24].
Proliferation marker Ki-67
Cells treated with IC50 concentrations of regorafenib, ATRA, and a sub-IC50 combination (100 × 5) were trypsinized and processed into a single-cell suspension. These cells underwent intracellular staining with an anti-Ki-67 antibody [25]. First, the cells were fixed with 4% paraformaldehyde at room temperature for 10 minutes and permeabilized for 30 minutes using 1% Triton-X. To prevent nonspecific binding, a 2% bovine serum albumin solution was used for blocking, followed by staining with a specific Ki-67-conjugated antibody. This staining process was conducted at 4°C for 60 minutes in the dark. The fluorescent intensity of the labelled cells was analysed using a Becton Dickinson FACS calibre flow cytometer operated with Cell Quest software.
Analysis of tumour biomarkers via sandwich ELISA technique
The levels of various proteins in Caco-2 and HCT-116 cell lysates or supernatants of different treatment groups were determined using the sandwich ELISA technique [26]. This method is highly specific and sensitive. The analysed biomarkers included NF-κB (MyBiosource, catalogue # MBS2514316), AMPK (MyBiosource, catalogue # MBS2514316), pERK (MyBiosource, catalogue # MBS2511875), and VEGF (CUSABIO, catalogue # CSB-E11718h). The analysis was performed following the manufacturer’s instructions, and the results represent the mean ± SEM of 3 separate experiments, each conducted in triplicate.
Caspase-3 activity
The activity of active caspase-3 was assessed using a colorimetric kit (Sigma, Product Code: CASP-3-C). Caspase activity was evaluated based on the spectrophotometric detection of the chromophore p-nitroaniline (pNA) at 405 nm after cleavage from its labelled substrate DVD-pNA. The concentration of the resulting cleaved pNA was determined using a calibration curve [27].
Statistical analysis of the data
The data obtained from the experiments were represented as the mean values with corresponding standard error of the mean (SEM). To assess the statistical significance of the results, a one-way analysis of variance (ANOVA) was employed, followed by a post hoc Tukey’s multiple comparison test. Graphical data representation and statistical analysis were carried out using Graph Pad Prism version 7.0, developed by Graph Pad Software Inc. (California, USA). In this analysis, statistical differences were considered significant when the p-value was less than 0.05.
Result
The effects of regorafenib and/or ATRA on cell viability in both HCT-116 and Caco-2 cells are illustrated in Figures 1 A–C. Both drugs displayed concentration-dependent cytotoxic effects. Regorafenib treatment significantly reduced the viability of Caco-2 and HCT-116 cells, with respective IC50 values of 8 µM and 7 µM. All-trans retinoic acid also inhibited cell growth with an IC50 of 110 µM in both cell lines. Additionally, the combination of ATRA and regorafenib exhibited enhanced antiproliferative activity compared to regorafenib alone in both cell lines.
Fig. 1
The effect of regorafenib (1–8 µg/ml) (A), all-trans retinoic acid (ATRA) (80–150 µg/ml) (B), and combinations of regorafenib and ATRA on HCT-116 and Caco-2 cells for 48 hours (C)
Data are represented as the mean ± standard deviation; each data point represented an average of 3 independent experiments (triplet).
P < 0.05 indicates a significant difference for regorafenib and/or ATRA vs. the control group in HCT-116 cells
P < 0.0001 indicates a significant difference for regorafenib and/or ATRA vs. the control group in Caco-2 cells
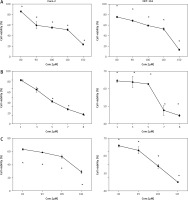
Ki-67, as a proliferation marker, revealed a significant reduction in cell proliferation compared to the control group in both the regorafenib- and ATRA-treated groups (Figs. 2, 3) (p < 0.05). Notably, the combination of regorafenib and ATRA showed a more pronounced decrease in cell proliferation when compared to the control group and to either the regorafenib- or ATRA-treated groups (p ≤ 0.0001) (Figs. 2, 3).
Fig. 2
The effect of regorafenib and/or all-trans retinoic acid (ATRA) on the proliferation of Caco-2 cells for 48 hours. Dot-plots from flow cytometric illustrating proliferation status in Caco-2 cells. Control group (A), cells treated with IC 50% of regorafenib (B), cells treated with IC 50% of ATRA (C), cells treated with sub Ic 50% of regorafenib and ATRA (D), and the total percentage of Ki-67 in Caco-2 cells treated with the indicated concentrations of regorafenib and/or ATRA for 48 hours (E)
All data are expressed as the mean ± standard deviation.
Statistically significant differences between groups are designated as a < 0.05 vs. control group, b < 0.05 vs. regorafenib group, and c < 0.05 vs. ATRA group.

Fig. 3
The effect of regorafenib and/or all-trans retinoic acid (ATRA) on the proliferation of HCT-116 cells for 48 hours. Dot-plots from flow cytometric illustrating proliferation status in HCT-116 cells. Control group (A), cells treated with IC 50% of regorafenib (B), cells treated with IC 50% of ATRA (C), cells treated with sub Ic 50% of regorafenib and ATRA (D), and the total percentage of Ki-67 in Caco-2 cells treated with the indicated concentrations of regorafenib and/or ATRA for 48 hours (E)
All data are expressed as the mean ± standard deviation.
Statistically significant differences between groups are designated as a < 0.05 vs. control group, b < 0.05 vs. regorafenib group and c < 0.05 vs. ATRA group.

In terms of phosphorylated AMPK (pAMPK), the levels of pAMPK significantly increased in the regorafenib-treated group compared to the control (p < 0.05). The ATRA group also showed a significant increase in pAMPK levels, but this effect was observed only in the HCT-116 cell line (Fig. 4 B). Notably, the sub-IC50 combination treatment resulted in a substantial increase in pAMPK levels compared to cells treated with either regorafenib or ATRA alone in both cell lines (p ≤ 0.0001) (Figs. 4 A, B).
Fig. 4
Effect of regorafenib and/or all-trans retinoic acid (ATRA) on phosphorylated activated protein kinase level in colorectal cancer cell lines. Caco-2 cell line (A), HCT-116 cell line (B)
All data are expressed as mean ± standard deviation of 3 separate experiments conducted in triplicate.
The statistical analysis was carried out using one-way ANOVA test followed by Tukey’s multiple comparisons test using GraphPad Prism software v 6.0.
Significant in comparison with control
Significant in comparison with IC 50 regorafenib-treated group (Reg)
Significant in comparison with IC 50 ATRA-treated group (p < 0.001)
Reg, regorafenib; comb, combination of sub-IC 50 of regorafenib and ATRA

Regarding annexin V, our results indicate a significant increase in cell apoptosis in the regorafenib-treated groups in both cell lines compared to the control group (p < 0.05) (Figs. 5, 6). Additionally, in the ATRA-treated group, a significant increase in apoptosis was observed, but only in the HCT-116 cell line (Fig. 6). Notably, the combination of regorafenib and ATRA resulted in a significant increase in cell apoptosis when compared to the control group and to either the regorafenib- or ATRA-treated groups (p ≤ 0.0001) (Figs. 5, 6).
Fig. 5
The effect of regorafenib and all-trans retinoic acid on the apoptosis of Caco-2 cells for 48 hours. Dot-plots from flow cytometric illustrating apoptotic status in Caco-2 cells. Cells in the upper-left quadrant are necrotic, cells in the upper-right quadrant have late apoptosis, the lower-left quadrant shows normal living cells, and the cells in the lower-right quadrant are early apoptotic cells (A–D), the total percentage of apoptosis in Caco-2 cells treated with the indicated concentrations of regorafenib and/or all-trans retinoic acid (ATRA) for 48 hours (E)
All data are expressed as the mean ± standard deviation.
Statistically significant differences between groups are designated as a < 0.05 vs. control group, b < 0.05 vs. regorafenib group, and c < 0.05 vs. ATRA group.

Fig. 6
The effect of regorafenib and all-trans retinoic acid on the apoptosis of HCT-116 cells for 48 hours. Dot-plots from flow cytometric illustrating apoptotic status in HCT-116 cells. Cells in the upper-left quadrant are necrotic, cells in the upper-right quadrant have late apoptosis, the lower-left quadrant shows normal living cells, and the cells in the lower-right quadrant are early apoptotic cells (A–D), the total percentage of apoptosis in HCT-116 cells treated with the indicated concentrations of regorafenib and/or all-trans retinoic acid (ATRA) for 48 hours (E)
All data are expressed as the mean ± standard deviation.
Statistically significant differences between groups are designated as a < 0.05 vs. control group, b < 0.05 vs. regorafenib group, and c < 0.05 vs. ATRA group.

Both Caco-2 and HCT-116 cells exhibited a significant reduction in VEGF levels (p < 0.05) following treatment with either regorafenib or ATRA, as well as with the combination therapy, compared to untreated control cells (Figs. 7 A, B). Notably, in Caco-2 cells, the combination treatment resulted in significantly lower VEGF levels compared to either regorafenib or ATRA treatment alone, underscoring the enhanced impact of the combination. In HCT-116 cells, the combination treatment also reduced VEGF levels compared to the control group, although the reduction was smaller than with regorafenib treatment alone.
Fig. 7
Effect of regorafenib and/or all-trans retinoic acid (ATRA) on vascular endothelial growth factor level in colorectal cancer cell lines. Caco-2 cell line (A), HCT-116 cell line (B)
All data are expressed as mean ± standard deviation of 3 separate experiments conducted in triplicate.
The statistical analysis was carried out using one-way ANOVA test followed by Tukey’s multiple comparisons test using GraphPad Prism software v 6.0.
Significant in comparison with control
Significant in comparison with IC 50 regorafenib-treated group (Reg)
Significant in comparison with IC 50 ATRA-treated group (p < 0.001)
Reg, regorafenib; comb, combination of sub-IC50 of regorafenib and ATRA

Examining another apoptosis marker, caspase-3, we observed a significant enhancement of caspase-3 activity (p < 0.05) in both cell types following ATRA and regorafenib treatment compared to the control group (Figs. 8 A, B). Notably, the combined treatment exhibited significantly higher caspase-3 activity than either the ATRA or regorafenib-treated groups in both cell lines (p ≤ 0.0001) (Figs. 8 A, B).
Fig. 8
Effect of regorafenib and/or all-trans retinoic acid (ATRA) on caspase-3 activity level in colorectal cancer cell lines. Caco-2 cell line (A), HCT-116 cell line (B)
All data are expressed as mean ± standard deviation of 3 separate experiments conducted in triplicate.
The statistical analysis was carried out using one-way ANOVA test followed by Tukey’s multiple comparisons test using GraphPad Prism software v 6.0.
Significant in comparison with control
Significant in comparison with IC 50 regorafenib-treated group (Reg)
Significant in comparison with IC 50 ATRA-treated group (p < 0.001)
Reg, regorafenib; comb, combination of sub-IC50 of regorafenib and ATRA

When compared to the untreated control group, both Caco-2 and HCT-116 cells exhibited a significant reduction in pERK levels (p < 0.05) upon treatment with either regorafenib, ATRA, or their sub-IC50 combination (Figs. 9 A, B). Notably, the combined treatment demonstrated significantly lower pERK levels than either regorafenib or ATRA alone (p ≤ 0.0001) (Figs. 9 A, B).
Fig. 9
Effect of regorafenib and/or all-trans retinoic acid (ATRA) on pERK level in colorectal cancer cell lines. Caco-2 cell line (A), HCT-116 cell line (B)
All data are expressed as mean ± standard deviation of 3 separate experiments conducted in triplicate.
The statistical analysis was carried out using one-way ANOVA test followed by Tukey’s multiple comparisons test using GraphPad Prism software v 6.0.
Significant in comparison with control
Significant in comparison with IC50 regorafenib-treated group (Reg)
Significant in comparison with IC50 ATRA-treated group (p < 0.001)
Reg, regorafenib; Comb, combination of sub-IC50 of regorafenib and ATRA

In both cell lines, a significant reduction in NF-κB levels (p < 0.05) was observed in cells treated with either regorafenib or ATRA, as well as their sub-IC50 combination (Figs. 10 A, B). Notably, the combined treatment resulted in significantly lower levels of NF-κB than either regorafenib or ATRA alone (p ≤ 0.0001) (Figs. 10 A, B).
Fig. 10
Effect of regorafenib and/or all-trans retinoic acid (ATRA) on nuclear factor kappa B level in colorectal cancer cell lines. Caco-2 cell line (A), HCT-116 cell line (B)
All data are expressed as mean ± standard deviation of 3 separate experiments conducted in triplicate.
The statistical analysis was carried out using one-way ANOVA test followed by Tukey’s multiple comparisons test using GraphPad Prism software v 6.0.
Significant in comparison with control
Significant in comparison with IC50 regorafenib-treated group (Reg)
Significant in comparison with IC50 ATRA-treated group (p < 0.001)
Reg, regorafenib; Comb, combination of sub-IC50 of regorafenib and ATRA
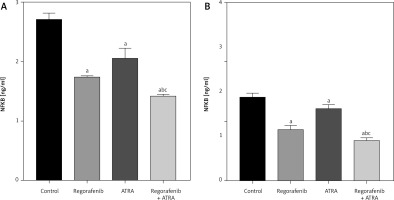
Discussion
The multifaceted nature of cancer development, entailing numerous molecular signalling pathways, underscores the need for extensive research in developing and enhancing the effectiveness of pharmacological drug combinations that target multiple oncogenic pathways. Our study was carried out to explore the combined effects of regorafenib and ATRA and to elucidate their effects on key signalling pathways implicated in CRC. We assessed the antitumour effects at both the cellular and molecular levels by evaluating cell viability and examining the levels of several crucial mediators in colorectal cancer signalling pathways, such as VEGF, ERK, NF-κB, Ki-67, AMPK, annexin, and caspase-3 activity.
Our findings align with previous studies that have demonstrated the ability of regorafenib to activate AMPK in NSCLC and HCC cells [28, 29]. This effect is significantly potentiated when regorafenib is combined with ATRA, which acts as an AMPK activator [30], providing a strong rationale for combining these drugs to suppress CRC cell proliferation. As established, AMPK functions as a metabolic tumour suppressor, regulating cell metabolism and growth [31] by creating a metabolic checkpoint in the cell cycle through its actions on mTORC1, p53, and other modulators [32]. Accumulating studies have confirmed the manifold anti-cancer effects of AMPK activation, including the initiation of autophagy, apoptosis induction, proliferation inhibition, metastasis suppression, immune system stimulation, and multidrug resistance reversal [33].
It is essential to note that ATRA plays a significant role in this study by activating AMPK through a unique mechanism, as demonstrated by Ishijima et al. [30]. All-trans retinoic acid was shown to inhibit the energy-producing pathway in cancer cells by downregulating the expression of key glycolysis genes GLUT-1 and LDHA. Our results indicate variations in the expression levels of important markers between control and treated cells. In untreated cells, higher gene expression levels of VEGF, ERK, NF-κB, and Ki-67 were observed, suggesting the natural activation of these oncogenic signalling pathways. Annexin and caspase-3 levels were low in untreated cells, in line with the baseline state of control cells that have not undergone damage or programmed cell death (apoptosis).
Notably, the level of NF-κB was significantly decreased in ATRA-treated cells due to its role as an AMPK activator. Activated mitogen protein kinase indirectly inhibits the NF-κB signalling pathway through mediators like SIRT1 or peroxisome proliferator-activated receptor co-activator 1 (PGC-1) [34]. SIRT1 deacetylates the lysine 310 of the NF-κB p65 subunit, reducing the transcriptional activity of NF-κB [35, 36]. Furthermore, our study shows that ATRA effectively suppresses VEGF, a key angiogenic marker, which inhibits the PI3K/p-Akt/mTOR/NF-κB cascade [24]. This inhibitory effect on VEGF is attributed to AMPK activation [37], in line with studies demonstrating that pharmacological activators of AMPK inhibit tumour angiogenesis [38–41].
Interestingly, the effects of the regorafenib-ATRA combination on VEGF levels were contrasting in the 2 cell lines studied. In the HCT-116 cell line, the combination treatment led to a significant reduction in VEGF levels compared to single treatments, suggesting a potential effect. However, the Caco-2 cell line displayed an unexpected increase in VEGF levels with the combination treatment when compared to single drug. This finding highlights the complexity of interactions between these agents and emphasizes the need for further research to understand the mechanisms responsible for these differential effects on VEGF regulation. This outcome may be attributed to the genetic diversity between the 2 studied human cancer cell lines and the type of kinetic activation [42].
Furthermore, in ATRA-treated cells, a significant reduction in the expression of activated ERK was observed. This finding is in line with the research conducted by Liu et al. in 2018 [43], which demonstrated that ATRA contributes to the protection against CRC development by suppressing the activation of the MAPK/ERK signalling pathway. Additionally, it has been documented that AMPK activation results in the deactivation of ERK1/2 by inhibiting the interaction between eEF2K and MEK1/2 [44].
As for cell proliferation, our investigations revealed a substantial decrease in Ki-67 levels in both Caco-2 and HCT-116 cells following ATRA treatment. This effect can be attributed to the inhibition of the ERK signalling pathway [45]. The inhibition of ERK prevents the activation of downstream transcription factors and cell cycle regulators, ultimately leading to a reduction in cell proliferation. This outcome is consistent with the findings of Lokman et al. in 2019 [46], who reported a decrease in Ki-67 expression in serous ovarian cancer tissues upon ATRA treatment.
Regarding apoptosis markers, our study assessed annexin levels (a marker of early apoptosis) and caspase-3 activity (a key enzyme in the apoptotic pathway). All-trans retinoic acid exhibited varying effects on annexin levels across different cell lines, with a more pronounced impact on caspase-3 activity, highlighting its potential in promoting apoptosis. Both ATRA and regorafenib treatments significantly elevated caspase-3 activity in both cell types compared to the control group. Notably, the combination of regorafenib and ATRA resulted in even higher caspase activity, indicating a substantial enhancement of cell apoptosis.
These findings emphasize the crucial role of ATRA in apoptosis induction by significantly increasing caspase-3 activity. The combination of regorafenib and ATRA demonstrates a significant enhancement of apoptosis, surpassing the individual treatments with either ATRA or regorafenib. These results align with previous studies that have demonstrated the ability of ATRA to induce apoptosis, inhibit cell proliferation, and activate the mitochondrial apoptotic pathway in HepG2 cells via AMPK activation [30].
Conclusions
Our study investigates the combined effects of regorafenib and ATRA on CRC cell lines, Caco-2 and HCT-116. We have demonstrated that this novel combination exerts significant antitumour effects at both cellular and molecular levels. Our findings suggest that ATRA plays a crucial role in suppressing cell proliferation and inducing apoptosis, while the combination of regorafenib and ATRA enhances these effects. The molecular analysis reveals that ATRA reduces the expression of VEGF, ERK, and NF-κB, possibly through AMPK activation, which contributes to the inhibition of oncogenic pathways. However, it is essential to note that these effects may vary between different cell lines, underscoring the genetic diversity in cancer. Our research underscores the potential of the regorafenib-ATRA combination as a promising strategy in CRC therapy. Further studies are needed to elucidate the underlying mechanisms and optimize the therapeutic application of this combination.