Introduction
Allergic skin diseases mainly include urticaria, atopic dermatitis (AD), allergic contact dermatitis (ACD), drug eruption and photosensitive skin diseases, etc. [1], the target organ that triggers these diseases is skin comprising mainly of keratinocyte (KC) and fibroblast (FBs). As an organ with composition complexity and multifunction, skin serves as the physical and chemical barriers for organism and shows stress response against external environment [2]. Specifically, inflammatory response will take place when then skin receives chemical, physical and immune stimulations, upon which many cytokines that affect the production, growth, differentiation and migration of inflammatory cells or the ones with activation effect, can be released. The allergic reaction of skin refers to anaphylaxis with high incidence, the allergic symptom generally appears firstly on the skin when external allergen stimulates the organism, which brings about a particularly serious effect on army officers and soldiers who perennially live and work in severe environments, such as frontier defence and islands. Therefore, it will be highly desirable to get in-depth understanding of the pathogenesis of allergic skin diseases for the development of new drugs.
Eosinophils (Eos) constitute the main effector cell of allergic diseases, which also serves as the main target cell in treating allergic diseases [3, 4]. In this regard, preventing Eos recruitment on allergic sites can help to prevent the occurrence of allergic inflammation, the factors that show chemotaxis effect on Eos has received extensive attention. The first discovered main chemotactic factor was IL-5, while the asthma model of mouse knockout by interleukin 5 (IL-5) showed that there remained Eos infiltration in the tissue [5], indicating that other important mechanisms may participate in this process. It was not until 1993 that eotaxin was discovered and its significance on Eos was also verified. A further study confirmed that IL-5 mainly related with the maturity and differentiation of Eos [6], while the main role of eotaxin was to initiate the chemotaxis process that recruits Eos onto inflammation site and maintains its subsistence. The combination of eotaxin and CC chemokine receptor 3 (CCR3) on Eos surface gives rise to a series of biological effects, such as activating Gi protein, temporarily elevating the concentration of calcium ion in the cell, cytoskeleton rearrangement, etc. Also, the transduction pathway of the MAP kinase signal is activated, which, together with transferrin, makes the acceptor molecules produce temporary invagination and endocytosis effects [7, 8]. This process makes Eos release toxic medium and further leads to tissue damage, thereby resulting in the occurrence and development of inflammation.
As a key chemotactic factor during Eos recruitment on allergic inflammation site, eotaxin has received wide attention, which is also regarded as one of the important therapeutic targets [9]. Nevertheless, current research focuses more on the pathogenesis of eotaxin in allergic diseases of the respiratory tract, there are also reports on its expression in some skin allergic diseases, such as AD, ACD, drug allergy, etc., while its expression rule and regulation mechanism are far from unambiguous and need in-depth understanding. In this work, on the basis of a mouse ACD model, together with the KC line HaCaT cell and FBs that constitute epidermal and corium layers, respectively, the expression status and sites, dynamic process and transcription mechanism of eotaxin are investigated in vivo and in vitro. The combined findings may lay theoretical foundation for designing therapeutic schedule using eotaxin as a target that helps for the treatment of skin allergic diseases.
Material and methods
Expression rule of eotaxin in the mouse ACD model
Animals were cared for in accordance with the Guide for the Care and Use of Laboratory Animals (1996, published by National Academy Press, 2101 Constitution Ave. NW, Washington, DC 20055, USA) and the use of animals was reviewed and approved by the animal care review committee at the First Affiliated Hospital of the Second Military Medical University. A BALB/c mouse was taken as a model animal, DNFB was smeared onto the mouse ear, sensitization and intensified sensitization were initiated at the first day and second day, respectively; activation was carried out at the fifth day to trigger a skin allergic response. After activation, the thickness of mice (n = 24) was measured at different time courses during 1–120 h. Also, one group with absence of 2,4-dinitrofluorobenzene (DNFB) acetone olive oil solution was prepared as the control group. The concentration of serum IgE before sensitization, 24 h after first sensitization (24 h) and second sensitization (24 h) as well as 24 h after activation were detected by enzyme-linked immunosorbent assay (ELISA). The kinetic process of swelling degree and inflammation were verified by the pathological examination results of skin tissue and blood inflammatory cell counts. Flow cytometry (FCM) was utilized to detect the T cell amount ratio of CD4+ to CD8+ in blood, the immunohistochemistry method was adopted to detect the amounts of CD4+ and CD8+ T cells in blood and tissue, which can well indicate the recruitment rules of the two cells. The pathological process and infiltration status of CD4+ and CD8+ T cells in the mouse ACD model, as well as the dynamic process of eotaxin secretion were reflected by the immunostaining results. The blood cell counts (n = 6) of mouse (Eos, neutrophils (Neu), lymphocyte (Lym), monocytes (Mon)) at different time courses after activation were also detected.
The construction of PUC57-eotaxin and PBS-eotaxin was conducted, accompanied by the electrophoresis and sequencing results to examine the validity of eotaxin probe sequence and quality.
The in situ hybridization (ISH) technique was used to detect the expression status (positive expression site) of eotaxin mRNA in the allergic skin of mouse, aiming to verify the main source of eotaxin under allergic status. Real-time polymerase chain reaction (PCR), immunohistochemical (ICH) and western-blotting methods were utilized to detect the kinetic rule of eotaxin in tissue, ELISA was used to detect the concentration of eotaxin in serum. Specifically, the real-time PCR relative quantitative method, together with OD260/280 examination, overall RNA electrophoresis results and dissociation curves were utilized to verify the integrity of RNA extraction and non-specific amplification of PCR products. The western-blotting method was further used to detect the expression of eotaxin (8.4 kD) in ear tissue of the mouse ACD model, the internal reference protein was glyceraldehyde phosphate dehydrogenase (GAPDH) (36 kD).
Regulation mechanism of eotaxin produced in skin cell
The HaCaT cell line and 6–10th generation FBs were adopted in this work. Microscope images show that HaCaT cells are ovals and polygons, with uniform sizes and high refractivity as well as clear boundary. The cell formed multiple big and small islets, distributing at the bottom of the culture dish. Abundant cytoplasm can be seen, the oval and rounded cell nuclear located near the cell centre, the cells showed inlaid arrangement with cobble-like structure but no mutual crossing. The distributed cell islets gradually expanded and linked together along with cell proliferation, which resulted in monolayer fusion after culturing for only 2–3 d. FBs showed fusiformis or long fibre structure, with a long and narrow cell body, clear boundary and abundant cytoplasm. The oval cell nucleus was located near the cytoplasm. The FBs arranged regularly and formed vortex-like or radial structure, which was fully distributed at the bottom of the culture dish along with cell proliferation. Fusion can be realized after culturing for only 2–3 d, as displayed in Supplementary Figure S1.
Tumour necrosis factor (TNF-α; 1 ng/ml, 10 ng/ml, 100 ng/ml) and IL-4 (2 ng/ml, 10 ng/ml, 50 ng/ml) were individually incubated with HaCaT cell line and FBs for 12 h, real-time PCR, FCM and ELISA methods were used to detect the expression status of eotaxin mRNA and protein. Specifically, the specific inhibitor ammonium pyrrolidinedithiocarbamate (PDTC) (100 µM) [10] of transcription factor NF-κB was added into the cell to realize joint incubation for 24 h, the two cells were then treated by TNF-α (100 ng/ml) for 12 h. As for eotaxin induced by IL-4 that regulates STAT6 phosphorylation, the transfection efficiency was evaluated by mixing siRNA with a different amount of the transfection reagent. The transcription factor STAT6 in KC and FBs was knockout by STAT6-specific siRNA (100 nM). After transfection for 36 h, IL-4 (10 ng/ml) was used to stimulate the cells for 12 h to observe the expression status of eotaxin mRNA and protein. To detect the effect of IL-4 on STAT6 phosphorylation in the two cells, IL-4 with different concentrations was prepared to stimulate the cells, upon which STAT6 (total STAT6, 94 kD) and phosphorylated STAT6 (pSTAT6, 100 kD) were detected at different time courses. Electrophoretic mobility shift assay (EMSA) and western-blotting methods were adopted to detect the nuclear translocation of transcription factor NF-κB and phosphorylation of STAT6.
Results
Expression rule of eotaxin in the mouse ACD model
ACD model establishment
Skin allergic symptom and swelling degree of mouse ear in the ACD model
After sensitization and activation using DNFB acetone olive oil solution, it was found that the ear tissue of the mouse turned red and swollen within 10 min, as shown in Figure 1 A. This situation got more serious but gradually mitigated after ~3 d, which recovered after 5 d and generally kept identical with that of the control group. The mouse ear showed no obvious exudation and ulceration, the scratching frequency was slightly higher than that of the control group. As shown in Figure 1 B, obvious swelling of the mouse ear occurred at 1 h after activation (immediate-phase response), which underwent a platform and kept increasing after 12 h (late-phase response), the peak value can be found at 24 h, as evidenced by the highest ear thickness of 0.65 ±0.07 mm. Accordingly, the swelling degree can reach up to 0.26 ±0.4 mm and last until 48 h, which decreased after 72 h and returned to normal status.
Serum IgE concentration and pathological observation of skin tissue in the mouse ACD model
The concentration of serum IgE before sensitization was 10.26 ±2.15 ng/ml, which was 94.89 ±16.75 ng/ml after second sensitization, apparently higher than that of first sensitization (58.29 ±10.41 ng/ml). A further increase in the concentration can be detected after activation (111.08 ±15.66 ng/ml). In addition, according to the staining results of H-E, it was found that large amounts of inflammatory cells in the mouse were infiltrated 1 h after activation, which increased with prolonging time while the inflammation turned better at 72 h, as shown in Figure 2. Among these, Eos (2–8 per HP) can be detected for the skin at different time courses after allergy, while they lacked regularity in different allergic reaction processes.
Blood cell counts in the mouse ACD model
As shown in Supplementary Figure S2, the Eos count was 0.09 ±0.04 × 107/l in the normal group. After activation, the Eos count in blood showed an obvious increase and reached the highest value of 6.63 ±1.12 × 107/l at 24 h, which then gradually decreased and recovered to the normal value. It was also found that the Neu, Lym and Mon decreased at different levels after skin allergy, which decreased with the alleviation of allergic progress and then recovered to the normal value. No basophilic granulocyte in blood was observed during the allergic process.
Infiltration in skin tissue and recruitment in blood of CD4+ and CD8+ T cells in the mouse ACD model
As displayed in Figure 3, the immunostaining results show that CD4 and CD8 molecules of the mouse ACD model skin presented dispersed distribution. With the occurrence and development of inflammation, CD4+ and CD8+ T cells increased at different levels, both of which recovered to normal values when the inflammation turned alleviated. The section in the negative control group with addition of the secondary antibody while absence of the primary antibody showed no positive staining, which proves that the secondary antibody did not contribute to non-specific staining.
Figure 3
Time course of CD4+ and CD8+ T cells infiltration in skin tissue of the mouse ACD model detected by IHC (100×)
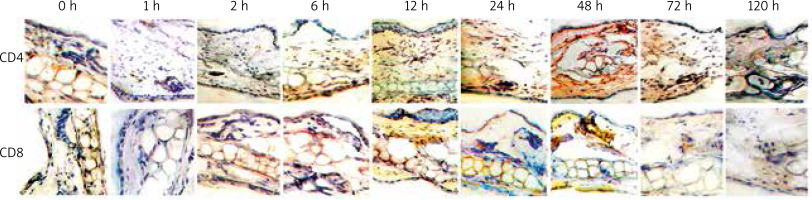
Figure 4 gives the recruitment rule of CD4+ and CD8+ T cells in the blood of the mouse ACD model, which shows a very similar dynamic rule with that of tissue. Specifically, CD8+ T cell increased rapidly at the initial stage of allergy, which presented downtrend earlier than that of CD4+ T cell. The amount of CD4+ T cell always accounted for a higher proportion, which was more obvious in blood than that in tissue and the increment amplitude was also larger. We also evaluated the variation of the ratio of CD4+ to CD8+ T cells during the occurrence and development of allergy, which gradually decreased at the initial stage and then increased, and finally, recovered to the normal value.
Occurrence site and dynamic rule of eotaxin in the mouse ACD model
Orientation of eotaxin expression in the tissue of the mouse ACD model
The construction of PUC57-eotaxin plasmid is shown in Figure 5 A, and the sequencing results are shown in Supplementary Figure S3. The electrophoresis map after plasmid extraction and Xho I/EcoRI double digestion is shown in Figure 5 B, the sequencing results of PBS-Eotaxin plasmid are shown in Supplementary Figure S4, the electrophoresis map after plasmid extraction and digestion is shown in Figure 5 C. The electrophoresis and sequencing results verified the correctness of Eotaxin probe sequence, and OD260/280 = 1.7–2.0, suggesting qualified purity.
Figure 5
A – pUC57-eotaxin plasmid construct map; Plasmid electrophoresis. B – pUC57-eotaxin plasmid, C – PBS-eotaxin plasmid. 1 – plasmid, 2 – plasmid digested by Xho I/Eco RI, M – maker; a – eotaxin plasmid, b – empty plasmid, c – eotaxin probe
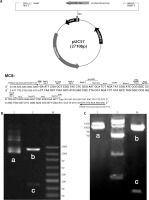
Based on the ISH experiment, the positive expression site of eotaxin mRNA in the allergic skin tissue of mouse is shown in Figure 6. Eotaxin mRNA expression was not detected in the normal mouse, probably due in large part to the low abundance. A few positive expressions around the endothelium of the blood vessel was detected 2 h after activation, a large expression in the dermal layer was detected at 6 h. The epidermal layer also showed expression and the amount reached the highest value at 12 h and decreased at 48 h, which generally recovered to the normal level after 72 h. Also, it can be clearly observed that eotaxin mRNA mainly expressed in the dermal layer, the expression amount in the epidermal layer was low, the expression can also be detected around the blood vessel and cartilage tissue. The negative control group without a probe showed no positive staining.
Kinetic expression process of eotaxin mRNA in the ear tissue of the mouse ACD model
The overall RNA electrophoresis results and real-time PCR dissociation curve verified the integrity of RNA extraction and non-specific amplification of PCR products. The sequencing of PCR products also confirmed that the amplified fragments were the specific fragment of eotaxin, as shown in Supplementary Figure S5.
The expression status of eotaxin mRNA in mouse ear was detected at different time courses after allergy, as shown in Supplementary Figure S6. Eotaxin expression can be detected 2 h after activation, which reached peak values at 6–24 h and decreased at 48 h and generally recovered to normal status at 72 h.
Dynamic process of eotaxin secretion in the ear tissue of the mouse ACD model
As observed from the staining results in the inflammation group, obvious eotaxin secretion can be detected when the mouse ear skin was under allergy for 6 h, which reached peak values at 12–24 h and decreased obviously at 48 h, almost no eotaxin expression can be detected at 72 h. Also, eotaxin mainly distributed around epidermis, dermis and cartilage, positive staining around the blood vessel was also detected, as shown in Figure 7. The negative control section with addition of the secondary antibody while absence of primary antibody showed no positive staining, thus verifying that the secondary antibody would not contribute to non-specific staining.
The expression of eotaxin (8.4 kD) in the ear tissue of the mouse ACD model using western-blotting method confirmed the dynamic rule of eotaxin secretion, the peak values appeared at 6–48 h (Supplementary Figure S7), which verified that the expression of eotaxin protein kept consistency with that of mRNA, this result also had an element of timing by taking mature blood inflow of Eos into consideration.
Eotaxin concentration detection of serum in the mouse ACD model
The concentration of eotaxin in serum of the mouse ACD model was detected at different time courses (n = 6), the result showed that eotaxin in plasma increased slightly, the maximum value appeared at 12 h (31.38 ±4.49 pg/ml), which was 13.52 ±2.84 pg/ml in the normal group, as shown in Supplementary Figure S8.
Regulation mechanism of eotaxin produced in skin cell
Does-effect between HaCaT cell induced by TNF-α/IL-4 and eotaxin expressed by FBs
The extracted cell RNA was of qualified purity, as evidenced by OD260/280 examination. The overall electrophoresis results of RNA and real-time PCR dissociation curve verified the integrity of RNA extraction, the PCR products showed no non-specific amplification. The sequencing result of PCR product also verified that the amplification section was exactly the specific section of eotaxin, as shown in Supplementary Figure S9.
TNF-α and IL-4 can realize the up-regulation of the eotaxin expression level and present dose-dependent characteristics. The basic secretion amount of eotaxin in HaCaT cell was 176.06 ±35.95 pg/ml, which increased to 734.65 ±206.80 pg/ml and 387.09 ±66.50 pg/ml after 100 ng/ml TNF-α and 10 ng/ml IL-4 induction, respectively. The basic secretion amount of eotaxin in FBs was 533.37 ±128.65 pg/ml, which increased to 4812.59 ±415.85 pg/ml and 2954.32 ±377.77 pg/ml after 100 ng/ml TNF-α and 10 ng/ml IL-4 induction, respectively. It is obvious that the capability of FBs in eotaxin secretion was obviously higher than that of HaCaT cell, and both cells showed basic expression of eotaxin, as shown in Figure 8.
Dynamic process of eotaxin expressed by HaCaT cell and FBs induced by TNF-α and IL-4 as well as the synergy between TNF-α and IL-4
The dynamic process of eotaxin production by HaCaT cell and FBs, which were individually or jointly induced by TNF-α (100 ng/ml) and IL-4 (10 ng/ml), was studied to explore the expression rule of eotaxin in the two cells and different stimulating factors. Figure 9 shows the expression status of eotaxin mRNA. FCM was used to detect the secretion rule of eotaxin protein, as shown in Supplementary Figures S10 and S11. The results further confirmed that eotaxin expressed in both cells and the capability of FBs in secreting eotaxin was superior than that of HaCaT cells, keeping good consistency with in vivo experiment. The overall findings supported that both HaCaT cells and FBs stimulated by TNF-α and IL-4 showed a similar rule in terms of eotaxin expression, which began at 1 h and reached peak values at 3–6 h and lasted for > 48 h. Afterwards, the amount of eotaxin expressed by FBs gradually decreased, while HaCaT cells can last for more than 72 h. Compared with FBs, HaCaT cells expressed eotaxin later, with a lower expression amount but longer duration time. In addition, after the two cells were individually or jointly stimulated by TNF-α (100 ng/ml) and IL-4 (10 ng/ml), ELISA was adopted to detect the expression status of eotaxin. The results showed that when both TNF-α and IL-4 were used, they produced a synergetic effect in terms of eotaxin secretion. As shown in Supplementary Figure S12, the secretion amount can reach high up to 4844.32 ±353.46 pg/ml and 28382.58 ±1491.48 pg/ml in HaCaT cells and FBs, respectively.
Figure 9
Eotaxin mRNA expression induced by TNF-α and/or IL-4 in two skin structural cells. N = 6, A – HaCaT cells, B – FBs; ■ TNF-α + IL-4, ♦ TNF-α, ▲ IL-4
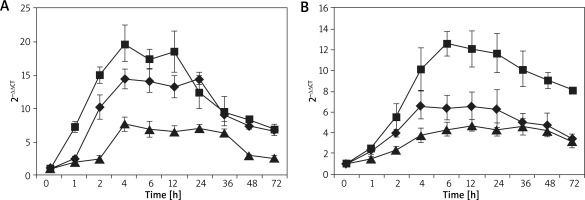
Transcription mechanism of TNF-α and IL-4 induced HaCaT cell and FBs that produced eotaxin
NF-κB nuclear translocation regulated by TNF-α to induce eotaxin production by the two cells
It was found that both eotaxin mRNA and protein were under obvious suppression of the two skin cells, the suppression rate can reach around 80% (Figure 10), which confirmed that the eotaxin expression in the two cells was regulated by the effect of TNF-α on NF-κB.
Figure 10
The inhibitory effect of TNF-α inducing eotaxin generation by PDTC in two skin cells. N = 6; A – Eotaxin mRNA expression, B – Eotaxin secretion; ■ FBs, ❑ HaCaT cells
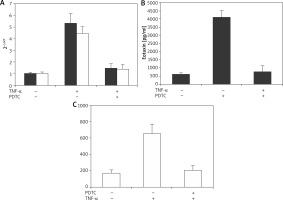
The dynamic process of NF-κB nuclear translocation regulated by TNF-α was further explored. Gel shift composite was observed in sequence-specific NF-κB during the EMSA experiment. Supplementary Figure S13 revealed a dose-dependent characteristic of NF-κB regulation by TNF-α. Also, the peak time for nuclear translocation of NF-κB in two cells was different, which was 30 min for FBs and 45 min for HaCaT cell. This result was in line with the kinetic process of eotaxin secretion induced by TNF-α. Eotaxin expression in HaCaT cell later than in FBs further verified that eotaxin secretion was affected by NF-κB nuclear translocation regulated by TNF-α.
NF-κB nuclear translocation regulated by IL-4 to induce eotaxin production by the two cells
The transfection efficiency of HaCaT cell increased with the increasing dose of the transfection reagent, which can reach up to higher than 80% under most conditions. However, when the ratio of the transfection reagent (μl) to NC siRNA-FAM (μg) increased to 12 : 5, obvious cytotoxicity appeared, despite the higher transfection efficiency of 90%. In this regard, when the ratio of transfection reagent (μl) to NC siRNA-FAM (μg) was 8 : 5, favourable transfection efficiency of 90% and low cytotoxicity can be simultaneously realized. As for FBs, the transfection efficiency of cell decreased with the increasing dose of the transfection reagent, favourable efficiency of >80% and low cytotoxicity can be obtained when the ratio of transfection reagent (μl) to NC siRNA-FAM (μg) was 6 : 2. Supplementary Figures S14 and S15 show the optimal transfection efficiency of the two cells.
The STAT6 mRNA knockout effect can be found in Supplementary Figure S16. The siRNA in the negative control group (siRNA) exerted no impact on the expression of the target gene, the effect of protein after knocking out the gene is shown in Figure 11.
Figure 11
STAT6 phosphorylation induced by IL-4 in two skin cells detected by western-blotting method
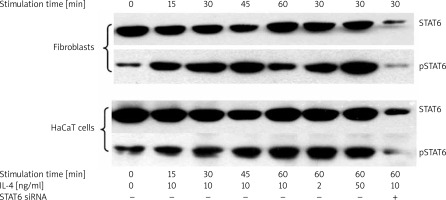
As shown in Supplementary Figure S17, after 36 h transfection using STAT siRNA and 12 h stimulation using 10 ng/ml IL-4, the expression of about 80% eotaxin was suppressed, which indicates that the regulation of IL-4 towards eotaxin was realized by inducing the phosphorylation of STAT6 and transcription.
The effect of IL-4 on the phosphorylation of STAT6 in the two cells was evaluated. As shown in Figure 11, the effect of IL-4 on STAT6 phosphorylation was dose-dependent, but not that obvious. In FBs, the peak value for STAT6 phosphorylation can be detected after only 30 min, which was 60 min for the HaCaT cell. This finding was consistent with the difference of IL-4 induced eotaxin expression in the two cells, which also confirmed that STAT6 siRNA can significantly suppress the protein expression of STAT6.
Discussion
Previous reports have proposed that DNFB can serve as half antigen to trigger ACD, which has become the classical model in ACD [11–16]. In this work, the mouse ACD model was adopted, along with the use of DNFB for sensitization to stimulate the allergic response of mouse skin. Based on the two main structural cells (HaCaT cell and FBs) that constitute the skin, the expression rule and regulation mechanism of eotaxin induced by TNF-α and IL-4 were evaluated in vitro. We determined the expression site of eotaxin in the skin of the mouse ACD model for the first time, and revealed the kinetic process of eotaxin expression in the allergic process, which further verified the certainty that KC can secrete eotaxin, as well as the common and different contributions of KC and FBs in secreting eotaxin.
After DNFB modelling, the thickness of the mouse ear, the infiltration status of inflammatory cells of mouse ear skin and the increased inflammatory cell amount in mouse blood were detected, upon which the mouse ACD model was successfully established. In addition, the variations of CD4+ and CD8+ T-cells in tissue and blood indicate that the body immunity status of the mouse changed obviously after skin allergy, which presented the kinetic rule, thereby revealing that the change of body immunity status can function as the indicator for the judgement and evaluation of the allergic reaction.
Encouraged by the above in vivo and in vitro experimental results, it is first acknowledged that eotaxin in allergic skin diseases mainly comes from FBs in the dermal layer. In the inflammatory skin of the mouse ACD model under the induction of TNF-α and IL-4, both the corium layer and FBs can express a large amount of eotaxin mRNA and protein, higher than that of the epidermal layer or KC. These findings verify that both KC and dermal FBs contribute to the eotaxin secretion. Being different from previous reports which adopted the ICH method and showed that eotaxin mainly came from the epidermis cell [17], the ISH method was utilized in this work to detect the eotaxin mRNA expression in the tissue, which can provide more precise localization, the results were further confirmed by in vitro cell experiment.
In the pathological tissue section, although the infiltration of Eos in the tissue was detected, the rule in different allergic time courses was not observed. According to previous reports [18, 19], there barely existed intact Eos when it infiltrated in the skin, the real process of Eos entering into the tissue can hardly be observed. Nevertheless, the increase of eotaxin can be regarded as the infiltration of Eos in tissue.
The IgE level in serum of the mouse ACD model was also detected. IgE was known as an important medium in the allergic reaction of mediated skin, which activates mast cells and DCs via IgE acceptor (FcεRI) with high affinity [20]. Also, the skin reaction mediated by IgE was also accompanied by a more obvious increase of Eos in tissue [19]. In this work, the increment process of IgE in serum was similar with other reports.
At the in vitro level, we verified that eotaxin expression can be induced by TNF-α and IL-4 via two transcription pathways, NF-κB and STAT6. Furthermore, TNF-α and IL-4 show strong synergy in terms of eotaxin expression.
There remain controversial comments on the effects of CD4+ and CD8+ T cells in the immune reaction. Some studies proposed that the ratio of CD4+ to CD8+ T cell (CD4/CD8) can address the immune status of the organism [21, 22]. Other studies pointed out that CD4+ and CD8+ T cells were mainly associated with allergic reactions and autoimmune diseases, respectively [23]. Also, some reports proposed that Th2-type reaction was mainly mediated by CD4+ T cell [24, 25], such as the secretion of IL-4 and IL-5; while CD8+ T cell was mainly related with the secretion of Th1-type cell factor [26], such as TNF-α and γ-IFN. Although CD4+ and CD8+ T cells played different roles in immune response reactions with different mechanisms, some of which remain undetermined, while their significance in the development and regulation of the immune reaction has been generally accepted [27–34].
In this work, the recruitment processes of CD4+ and CD8+ T cells in tissue and blood were investigated in the mouse ACD model, they showed similar tendencies. It was found that the two T cells behaved in different ways in skin allergic reactions and related closely with reaction processes. First of all, CD4+ T cell showed an overwhelming advantage throughout the process, while CD8+ T cell increased earlier in tissue and blood after allergy, followed by CD4+ T cell. Similarly, CD8+ T cell decreased earlier than CD4+ T cell in a weakened allergic process. Therefore, CD4/CD8 firstly decreased and then increased and decreased again along with the symptomatic relief. Our experimental results suggest that CD8+ T cell is related with the allergic reaction while CD4+ T cell is related with the immune regulation during the allergic process.
Along with these experimental results, we deduce that the procedures related with Eos in skin allergic diseases can be as follows. Specifically, when the organism contacts with an allergen for the first time, it produces an allergen-specific IgE antibody; when the allergen stimulates the epidermic cells of skin, the immune system of the organism can be mobilized, allergen-specific IgE combines with activated mast cells and FcεRI on the surface of dendritic cells, which produces an immune reaction with a cascade amplification effect and results in the infiltration of Th cell into the tissue. Afterwards, the amounts of inflammatory cells including T cell, mast cell and macrophage increase and a large amount of cell factors are released, such as TNF-α, IL-4 and IL-5. These results further lead to the release of eotaxin in the structural cell of skin, accompanied by the differentiation of Eos precursor cell in marrow into mature Eos that further enters the blood. Eos enters the tissue under the chemotaxis of eotaxin, eotaxin in tissue combines with CCR3 acceptor on Eos surface to activate Eos and release toxic protein, resulting in tissue damage. Meanwhile, the existence of eotaxin prolongs the survival time of Eos, eotaxin can also be released after Eos activation in tissue, upon which the allergic reaction can be continued. Both CD4+ and CD8+ T cells first increased and then decreased during the different stages in the allergic reaction, which regulate the origin, occurrence and termination processes. With the disappearance of external stimulation factors and the effect of immune regulation, the allergic reaction turns gentle and finally finishes.
In this work, we also found that basic expression of eotaxin exists both in vivo and in vitro, indicating that eotaxin not only relates to allergy and inflammation, but also plays a role in innate immunity. In addition to the function in the chemotaxis of inflammatory cells, a few studies also reported other functions of eotaxin, such as its relationship with lung development [35], weak stimulation effect on KC growth [36], and angiogenesis effect [37]. That is, besides its relationship with pathological processes, eotaxin also relates with physiological processes, which remain to be explored.
In recent years, with the in-depth development of immunology and interdiscipline, more and more facts verified that the immune system affects the operation of nervous and endocrine systems via immunoregulators, such as IL and TNF. The nervous system can affect the functions of immune and endocrine systems via neurotransmitters. The endocrine system can control the activities of nervous and immune systems via various hormones. The three systems regulate each other, their common active substance and acceptor constitute the bridge for interconnection and information transfer channel, forming a complicated “immune-nervous-endocrine network” to regulate the physiological and pathological functions of the skin, including the regulation of cell growth, differentiation, immunity, inflammation and tissue repair [38–43]. In dermatology, the role of the “immune-nervous-endocrine network” in skin diseases has received increasing attention. Based on the structural cells of skin, skin participating into the key steps of the immune reaction is verified in this work, while its roles in physiological and pathological processes need further exploration.
Conclusions
In this work, the mouse ACD model was established, investigation and evaluation were carried out based on the ear swelling degree, pathological variation of skin tissue, IgE occurrence, inflammatory cell amount in blood, and the recruitment of CD4+ and CD8+ T cells in tissue and blood. It is verified that both epidermal and dermal layers in the mouse ACD model can express eotaxin, while the main source is the dermal layer. The dynamic process of eotaxin expression was also investigated, the results showed that eotaxin expressed 1–2 h after allergy and reached the peak value at 24 h and then gradually weakened, which generally recovered to the normal expression level after 5 d. The regulation mechanisms of eotaxin secretion in epidermal KC and dermal FBs were also verified. Specifically, TNF-α and IL-4c induce the eotaxin expression via two pathways, that is, NF-κB and STAT6.