Introduction
Metal nanoparticles are considered to be efficient in various biomedical applications as compared to other nanomaterials (Azharuddin et al., 2019). Biological synthesis of nanoparticles has been considered as an eco-friendly and natural approach (Ingale and Chaudhari, 2013), wherein herbal/medicinal plants have been used widely. Given its rapid, environmental-friendly, economical, and precise one-step approach, the fabrication of metal nanoparticles using plant extracts has gained significant attention (Ahmed et al., 2016). Various phytocompounds such as polysaccharides, alkaloids, tannins, phenolics, saponins, and terpenoids are used in the salt reduction method for nanoparticle synthesis (Ahmed et al., 2016). Plant metabolites are excellent reducing agents and thus exhibit the potential to convert the salt into nanoparticles (Mittal et al., 2013). Furthermore, the phytosynthesis and purification of nanomaterials are simpler than other methods (microbial- and/or chemical-mediated) (Mittal et al., 2013). For instance, the leaf extract of Sesbania aegyptiaca has been used to synthesize PEGylated silver nanoparticles, and its immunomodulatory and antioxidant properties were demonstrated in vitro (Pandian et al., 2018). Moreover, the synthesis of plant-based nanomaterials also offers good biocompatibility and fabrication of applicable nanomaterials (Borase et al., 2014).
Interestingly, various parts of plants have been used for nanoparticle synthesis (Borase et al., 2014), including leaf extracts of Combretum erythrophyllum (Jemilugba et al., 2019), stem extracts of Phyllanthus pinnatus (Balachandar et al., 2019), root extract of Berberis vulgaris (Behravan et al., 2019), and fruit extracts of Phyllanthus emblica (Masum et al., 2019). Quercetin is a widely distributed natural bioflavonoid in various medicinal plants (Bakova and Kolesarova, 2020). It possesses antioxidant, anti-inflammatory (Lesjak et al., 2018), anticancer, and neuroprotective (Dajas, 2012) properties. It has also been proven as a potential reducing agent in the synthesis of therapeutic nanomaterials (Lakshmi and Kim, 2019).
The stability of synthesized nanomaterials is influenced by the concentration of salt (core material) and reducing agents (Virkutyte and Varma, 2011). In this sense, various parameters have been used to optimize nanomaterial synthesis, including the reducing material (Ag+ to Ag0) (Pandian et al., 2010), salt concentration, and physical parameters (pH and temperature); these factors together influence the size and shape of nanoparticles (Nikaeen et al., 2020). For instance, many studies that adopted various pH values concluded that pH 7 is optimal for the synthesis of AgNPs (Bawaskar et al., 2010; Krishnaraj et al., 2012; Rajan et al., 2015). Eventually, temperature between 25 and 40 °C was considered suitable for the synthesis of AgNPs (Bawaskar et al., 2010; Saxena et al., 2016).
Given the nontoxic characteristic of biogenic metal nanoparticles (Seralathan et al., 2014), it was widely used in various biomedical applications (Singh et al., 2017). AgNPs are advantageous because of their unique properties such as optical (Evanoff Jr and Chumanov, 2005), electronic (Chen et al., 2009), biosensing (Lin et al., 2009), antibacterial (Ren et al., 2019), antioxidant (Taha et al., 2019), and anticancer (Anandan et al., 2019) properties. In particular, colloidal silver has been widely chosen for therapeutic applications as compared to other forms of nanosilver (Wei et al., 2015).
The synthesized nanoparticles are characterized using various instrumentation methods, and their biomedical efficacy is established. In line with this, MgO nanoparticles were synthesized using aqueous leaf extracts Pisidium guvajava and Aloe vera, and characterized by UV-VIS spectroscopy, Fourier-transform infrared spectroscopy (FT-IR), X-ray powder diffraction (XRD), field emission scanning electron microscopy (FESEM), energy-dispersive X-ray spectroscopy (EDAX), and X-ray photo-electron spectroscopy (XPS) (Umaralikhan and Jaffar, 2018). The antibacterial activity of the MgO nanoparticles was also studied. The results revealed that 5 mg/ml of MgO nanoparticles were effective in inhibiting 88% bacterial growth (Umaralikhan and Jaffar, 2018). Silver and gold nanoparticles were fabricated using the extract of Phoenix dactylifera, and the physicochemical properties were further characterized (Banu et al., 2018). Their anticancer activity on the MCF-7 breast cancer cell line was tested, which revealed a dose-dependent cytotoxicity by enhancing the expression of the antiapoptotic protein Bcl-2 and the proapoptotic protein p53 (Banu et al., 2018). The antioxidant properties of AgNPs synthesized with the extract of Lavandula stoechas were determined by (Mahmoudi et al., 2020). The synthesized AgNPs were then proved to exhibit antioxidant (125 μg/ml), antibacterial (125 μg/ml), and cytotoxic (500 μg/ml) properties in vitro (Mahmoudi et al., 2020).
In the present study, AgNPs were synthesized by the rapid one-pot method by using quercetin, a flavonoid found in many plants and red wine. The synthesized nanoparticles were then characterized by UV-VIS spectrophotometry, scanning electron microscopy (SEM), and transmission electron microscopy (TEM). The in vitro antioxidant potential of quercetin-functionalized NPs was also demonstrated.
Materials and methods
Materials
Quercetin (Q4951-10G) was purchased from Sigma-Merck, Bengaluru, India. All other chemicals were obtained from Hi-Media Laboratories Pvt. Ltd., Mumbai, India.
One-pot synthesis of AgNPs
The stock solution of quercetin (2 mM) was prepared using 1 mM sodium hydroxide solution at pH 8 and stored at room temperature. Next, 1 mM of 10 ml silver nitrate was prepared, to which 0.1 ml of quercetin solution was added in a drop-wise manner under constant stirring at room temperature. This facilitated the bio-reduction of silver ions (Ag+) to neutral ions (Ag0) in the solution, which was evidenced by the appearance of brown color. The resultant colloidal AgNPs were filtered through a 0.22-micron syringe filter and stored in refrigerator (2–8°C) until further analysis. The experiment was repeated three times, and the reliability of this method was confirmed.
UV-VIS spectrophotometry
The biosynthesis and reduction of silver ions to AgNPs were initially characterized on the basis of their absorbance spectra by a UV-VIS spectrophotometer (JASCOV-650 spectrophotometer) between the range of 300-700 nm.
SEM analysis
The size and morphology of the synthesized AgNPs were determined using a scanning electron microscope (Carl Zeiss EVO 18) at an accelerating voltage of 20 kV. The nanoparticle solution was diluted 5-fold with deionized water. Subsequently, a few drops of dispersed AgNPs were placed on an aluminum stub, which was airdried at 60° C for 5 min. The sample was then scanned, and photomicrographs were captured.
TEM analysis
The biosynthesized AgNPs were morphologically characterized by a transmission electron microscope (JEOL model 2100, Japan). The microscope was operated at an optimum accelerating voltage of 200 kV. A few drops of re-dispersed AgNPs were placed on a carbon-coated copper TEM grids and allowed for 10 min to settle down, followed by observation under the microscope.
FT-IR
The integrity of functional groups (–COOH, OH, –CH3, and –CO of quercetin) in quercetin and the bio-synthesized AgNPs was analyzed using a Fourier-transform infrared spectrophotometer (Shimadzu IR Tracer-100). One milligram of lyophilized quercetin and AgNPs was individually mixed with FT-IR grade KBr and subjected to hydraulic press to prepare a round disc. Sub-sequently, the discs were used in the spectrometer, in which the spectral ranges were set between 400 and 4000 cm–1.
Antioxidant activities of AgNPs
DPPH radical scavenging assay
The antioxidant potential of the synthesized AgNPs was estimated using the DPPH radical scavenging assay (Arora and Chandra, 2011). Briefly, 0.5 ml of various concentrations of AgNPs (10, 20, 40, 80, and 160 μg/ml) was mixed with 1 ml of 0.1 mM DPPH (2,2,1-diphenyl-1-picrylhydrazyl) solution in ethanol, followed by vigorous shaking and incubation at 37° C for 30 min (A2), which facilitates the formation of yellow color. Initially, the absorbance values of all the samples were measured and recorded before the reaction started. The absorbance of the decolorized DPPH solution was measured at 517 nm. The DPPH reducing activity of the AgNPs was calculated based on the difference between A1 and A2 absorbance values. For control, the antioxidant activity of ascorbic acid was determined by the DDPH assay at the concentration of 160 μg/ml, and the value was assumed as 100%. The experiment was repeated thrice, and the antioxidant activity was expressed as ascorbic acid equivalent.
Reducing Power Measurement Assay
Briefly, 0.1 ml of 1% (w/v) potassium ferricyanide and 0.1 ml of the synthesized AgNPs at various concentrations (10, 20, 40, 80, and 160 μg/ml) were mixed and incubated at room temperature for 30 min. To this mixture, 10% TCA (trichloroacetic acid) and 0.1% (w/v) ferric chloride were added and mixed thoroughly. This reaction mixture was incubated at room temperature for 20 min, and the absorbance was recorded at 665 nm in a spectrophotometer. For control, the antioxidant activity of ascorbic acid was determined at the concentration of 160 μg/ml, and the value was assumed as 100%. The experiment was performed thrice, and the antioxidant activity was expressed as ascorbic acid equivalent.
Ferric reducing antioxidant power (FRAP) Assay
The FRAP reaction mixture was prepared by adopting the protocol of Arora and Chandra, (2011). The mixture contained 300 mM acetate buffer (pH 3.6), 10 mM 2,4,6-tripyridyl-s-triazine (TPTZ), and 20 mM ferric chloride in a ratio of 10 : 1 : 1. For the FRAP assay, 0.5 ml of AgNPs (10, 20, 40, 80, and 160 μg/ml) were mixed with 2 ml of FRAP reagent and 1 ml of distilled water and incubated at room temperature for 10 min. After incubation, the formed blue color complex (Fe2+/TPTZ (2, 4, 6-tripyridyl-s-triazine)) was measured at 595 nm. Ferrous sulfate solution (1 mg/ml) was used as blank, and the antioxidant potential of the sample was determined by comparing the absorbance of the sample with that of the stock solution. For control, the antioxidant activity of ascorbic acid was determined by the FRAP assay at the concentration of 160 μg/ml, and the value was assumed as 100%. The experiment was performed thrice, and the antioxidant activity was expressed as ascorbic acid equivalent.
Results and discussion
Synthesis and characterization of AgNPs
In this study, quercetin-mediated synthesis of AgNPs was demonstrated by the rapid one-pot method. The synthesized nanoparticles were characterized by UV-VIS spectroscopy, FT-IR, SEM, and TEM. As previously shown, AgNPs exhibit antiviral (Lara et al., 2010; Morris et al., 2019), antibacterial (Behravan et al., 2019), antioxidant and antitumor (AlSalhi et al., 2019; Anandan et al., 2019), and immunomodulatory (Pandian et al., 2018) properties. Despite many available procedures to synthesize AgNPs (physical, chemical, and biological), the biological method holds great promise. It is comparatively efficient and nontoxic, thus making it better than other methods (Seralathan et al., 2014; Chowdhury et al., 2017; Tripathi et al., 2019). It is interesting to note that AgNPs synthesized using plant extracts that contain bioactive compounds such as flavonoids, alkaloids, saponins, steroids, and tannins exhibit superior pharmacological activity (Kuppusamy et al., 2016). In the present study, AgNPs were synthesized using quercetin, (a plant-derived flavonoid) (Bakova and Kolesarova, 2020) by the rapid one-pot method. A promising color change (from transparent to dark brown) indicated the formation of AgNPs, specifically colloidal AgNPs. UV-VIS spectrum is mostly adopted to confirm the synthesis and stability of NPs in aqueous solutions. Figure 1 shows the absorption spectrum of AgNPs and their unique surface plasmon resonance (SPR) intense peak at 420 nm, which was similar to that reported in our previous study (Pandian et al., 2018). A recent study (Lakshmi and Kim, 2019) reported quercetin-mediated synthesis of dual-functional gold nanoclusters for application in anticancer treatment and bioimaging. The size of the gold nanoclusters was reported as 1–3 nm with homogenous distribution (Lakshmi and Kim, 2019). Quercetin is a plant bioflavonoid (Bakova and Kolesarova, 2020) and is a potent reducing agent (Lakshmi and Kim, 2019) with antioxidant activity. It has also been proven as a potential phytocompound for the synthesis of therapeutic metal nanoparticles (Lakshmi and Kim, 2019). Vanaraj et al. (2017) reported the average size of quercetin-based AgNPs as 65 nm in diameter. Interestingly, the AgNPs synthesized in our study exhibited a size of 20 to 30 nm (Fig. 2) with uniformly spherical shape. TEM results revealed that the AgNPs were distinct with no aggregation (Fig. 3). Ahmed and Ikram (2015) reported one-step synthesis of AgNPs with Terminalia arjuna extract and measured the average size of the synthesized AgNPs as 50 nm. Atrocarpus altilis leaf extract was used to synthesize spherical-shaped AgNPs, and the average particle size reported was 34 nm (Ravichandran et al., 2016). Indeed, we have previously shown (Pandian et al., 2018) that AgNPs and PEGylated AgNPs synthesized using leaf extract of Sesbania aegyptiaca were between 16 and 23 nm and between 25 and 28 nm in size, respectively. The synthesized AgNPs were also proved to show immunomodulatory and anticancer activity in vitro(Pandian et al., 2018).
Functional group analysis with FT-IR
The wave numbers of FT-IR represent various functional groups of both AgNPs and quercetin. FT-IR was applied to analyze the functional groups of reduced metal nanoparticles and biomolecules. In a previous study, FT-IR revealed the functional groups and interactions of metal nanoparticles and biomolecules (Kunjiappan et al., 2014). The FT-IR spectra of quercetin, silver nitrate, and AgNPs are presented in Figures 4, 5, and 6, respectively. The names of the functional groups of quercetin, silver nitrate, and AgNPs are presented in Tables 1, 2, and 3, respectively. FT-IR analysis of AgNPs showed bands at 3439, 2360, 2365, 2881, 1649, 1471, 1251, 1103, and 950 cm1. The peaks at 1471 and 1251 cm–1were reported to be aldehydic–C-H stretching and alcoholic–C–O stretching, respectively (Balogun et al., 2015). It was also observed that the conversion of broad ligands (−OH) to soft ligands (−C−O) after the reduction process created a feasible environment for soft metal (Ag) to form co-ordination with soft carbonyl ligands (Chowdhury et al., 2014). Therefore, we attribute the reduction potential to quercetin, which was responsible for the reduction of silver nitrate to form AgNPs. This finding is in agreement with a recent study, which reported quercetin as a potential reducing agent for the synthesis of nanomaterials (Lakshmi and Kim, 2019).
Fig. 6
FT-IR spectra of silver nanoparticles; the functional groups of silver nanoparticles and quercetin (reducing agent) are presented
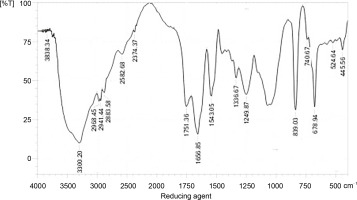
Table 1
The functional groups of quercetin as revealed in FT-IR
Table 2
The functional groups of silver nitrate as observed in FT-IR
Table 3
The list of functional groups found in quercetin-functionalized AgNPs
Antioxidant activity of quercetin-mediated synthesized AgNPs
AgNPs synthesized using various bioflavonoids exert antioxidant activities (Chowdhury et al., 2014; Salari et al., 2019). In the present study, the antioxidant activity of quercetin-functionalized AgNPs was studied in vitro by DPPH, FRAP, and reducing power measurement assays (Fig. 7A–C). The free radical DPPH is decolorized in the presence of antioxidants (Hasanuzzaman et al., 2013), which hold an unpaired electron that exhibits absorbance at 515 to 517 nm (Ahmad et al., 2020). We found a gradual increase in the antioxidant activity of AgNPs up to 80 μg/ml; however, the activity remains unchanged at 160 μg/ml. The antioxidant activity of AgNPs at 80 (μg/ml was observed as equivalent to 110, 66, and 69%, by DPPH, FRAP, and reducing power measurement assays, respectively. The antioxidant activity of ascorbic acid (control) was determined at the concentration of 160 μg/ml, and the value was assumed as 100%. The results of antioxidant activities were projected with ascorbic acid equivalence as demonstrated by previous scientific studies (Ahmad et al., 2020; Soltanabad et al., 2020). These results demonstrate a dose-dependent antioxidant activity of quercetin-functionalized NPs. The assays that we adopted to measure the antioxidant status have been widely used by other researchers (Ahmad et al., 2020; Soltanabad et al., 2020). The same assays were used to prove the antioxidant activities of apple fruit extracts and 2-phenoxypyridotriazolo pyrimidines in vitro (Abuelizz et al., 2020; Sethi et al., 2020).
Fig. 7
In vitro antioxidant activity of AgNPs determined by A) DPPH, B) FRAP, and C) power reducing assays
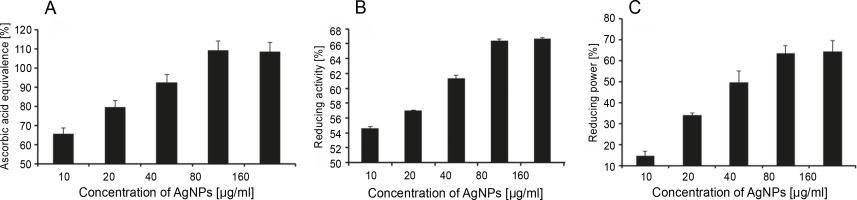
Several studies have confirmed the antioxidant and anticancer potential of AgNPs under in vitro and in vivo conditions (Sre et al., 2015; Salehi et al., 2016; Khorrami et al., 2018; Mousavi et al., 2018). In the present study, we also demonstrated that the synthesized AgNPs act as potential antioxidant molecules, specifically at the concentration of 80 μg/ml. The antioxidant, antibacterial, and cytotoxic effects of Piper longum fruit-based AgNPs were also proved in vitro (Reddy et al., 2014). It was further revealed that the antioxidant capacity of AgNPs determined by DPPH and reducing power assays differed due to various concentrations of AgNPs (100 to 600 μg/ml) (Reddy et al., 2014). Ravichandran et al. (2016) reported the antioxidant activity of AgNPs synthesized using Atrocarpus altilis at the concentration of 51.17 μg/ml (Ravichandran et al., 2016). Similarly, Cassia tora leaf extract-mediated single-step green synthesis of AgNPs, and their antioxidant activity was reported by Saravanakumar et al. (2015). The antioxidant role of AgNPs was studied in a concentration-dependent manner (12.5–200 μg/ml) by the DPPH scavenging assay with vitamin C as the standard (Saravanakumar et al., 2015). Taken together, all these results proved that AgNPs synthesized using plant sources have potential antioxidant properties, which are perhaps enhanced by the synergistic activity of the phytocomponents present in plant extracts. We therefore suggest that quercetin used in the present study could have influenced the reduction potential of silver and aided in the formation of AgNPs, which eventually culminates in the exhibition of antioxidant property.
Conclusions
In the present study, quercetin was used to synthesize AgNPs by the one-pot method. The synthesized AgNPs were 20–30 nm in size and spherical in shape. The significance of this work lies in the fabrication of AgNPs by using a single-step method and its extended stability at room temperature. The synthesized AgNPs were demonstrated to exert potential antioxidant properties in vitro. We conclude that phytochemical-mediated synthesis of metal NPs would be advantageous because it provides extended stability and synergistically improves their pharmacological properties.