INTRODUCTION
Inflammation of the airways is the fundamental feature of asthma pathophysiology. According to the guidelines, both the diagnosis and follow-up of patients with asthma are based on clinical and lung function parameters [1]. Research aimed at finding biomarkers of the disease process is gaining increasing interest. The objective of the studies published so far was to identify biomarkers of this phenomenon. Many of them have already been identified. However, their clinical relevance still remains not well established [2]. There are data suggesting that the pathomechanism of allergic diseases and asthma begins in the epithelium. The epithelium is an immunologically active tissue, which plays an important role in allergic reactions. The airway epithelium is the first line of defense against allergens, microbes and noxious agents [3, 4]. The evaluation of airway inflammation in asthma is based on complicated and invasive methods – bronchial biopsies and bronchial alveolar lavage – and on non-invasive ones, such as fractional exhaled breath condensate [5]. It seems appropriate to establish the clinical applicability of the biomarkers detected in exhaled breath condensate, as a method of evaluating ongoing inflammation in the bronchial tract that is easy to use in a clinical setting. The research aimed at identification of different biomarkers in EBC has gradually advanced. Similarly to blood, urine, sweat or tears, EBC may be thought as a valuable biological material, in which different biomarkers can be detected [6]. Oxidative stress plays an important role in the development of inflammation. The imbalance between the oxidative process and the anti-oxidative defense system initiates and is responsible for the maintenance of the inflammation, which in turn can cause cellular toxicity and cell death [7]. In that context, it is worth noting that both resident and inflammatory cells playing a role in asthma pathophysiology are producers and concomitantly targets of oxidant stress.
Reactive oxygen species (ROS) are produced by activated eosinophils, neutrophils, monocytes and macrophages as well as epithelial cells, smooth muscle, endothelium and fibroblasts [8]. The increasing generation of ROS plays a critical role in the development of airway inflammation. Increased cells’ exposure to ROS and depletion of defensive endogenous antioxidant systems lead to the increased susceptibility of cells to oxidative stress and the progressive loss of normal cell function [9]. To determine the oxidative status of an organism, it is necessary to determine the relationship between oxidant and antioxidant parameters in the body. Since lipid peroxidation is one of the most predominant and well-known consequences of intensified generation of free radicals, oxidative stress evaluation can be based on the concentration of the main product of lipid peroxidation – malondialdehyde (MDA). The other pro-oxidative parameters are: total oxidant status (TOS), lipofuscin and lipid peroxides (LPH). The largest role in the antioxidant activity of the body is played by enzymes such as total superoxide dismutase (SOD) and its isoenzymes: cytosolic (CuZnSOD) and mitochondrial (MnSOD), catalase (CAT), and glutathione peroxidase (GPx), reductase (GR) and S-transferase (GST). Other important anti-oxidative parameters that could be detected are: total antioxidant capacity (TAC), sulfhydryl groups (-SH) and ceruloplasmin [10].
The results of some studies have proved that the plasma levels of the anti-oxidant enzymes are decreased in patients with asthma [11, 12]. The results of both in vitro and animal studies give evidence that reactive oxygen species reproduce the characteristics of asthma. The weakened anti-oxidant defense system in peripheral blood in patients with asthma has been observed, but little is known about the function of that system in the lung cells of asthmatics [13].
AIM
The objective of our study was to estimate the oxidative stress parameters in blood serum, erythrocyte hemolysate and in exhaled breath condensate, in patients with moderate uncontrolled asthma (allergic and non-allergic), and to assess whether the intensity of that process may reflect the differentiation of asthma phenotypes.
MATERIAL AND METHODS
Forty-four patients (9 males, 35 females, age range: 35–59 years, mean: 47) with chronic moderate asthma (diagnosed according to the GINA criteria (1)) were included in the study. The diagnosis was based on clinical history, physical findings and lung function tests. The patients were divided into two groups. The first group consisted of 31 patients with moderate allergic asthma. The second group consisted of 13 patients with moderate non-allergic asthma. The patients controlled their asthma using inhaled corticosteroids in low doses (200–500 μg of beclomethasone/200–400 μg of budesonide/100–250 μg of fluticasone propionate/200 μg of mometasone furoate), low doses of inhaled corticosteroids in combination with β2-mimetics and anti-leukotrienes (10 mg of montelukast). None of them had used systemic corticosteroids within the 3 months prior to the study. The patients included in the study did not suffer from any other chronic diseases or inflammatory conditions (Table 1).
TABLE 1
Patient characteristics (asthma patients n = 44)
The diagnosis of atopy (differentiation between allergic and non-allergic asthma) was based on skin prick test results with inhalant allergens (Allergopharma diagnostic tests) and total IgE serum level evaluation. The skin prick tests were performed according to the EAACI guidelines [14]. In blood serum, erythrocyte hemolysate and in exhaled breath (EBC) condensate the following parameters were estimated: superoxide dismutase activity (SOD) and its isoenzymes (MnSOD, CuZnSOD), protein sulfhydryl groups (SH), catalase activity (CAT), glutathione reductase activity (GR), glutathione S transferase activity (GST), glutathione peroxidase activity (GPx), malondialdehyde (MDA), total oxidation status (TOS), total antioxidant capacity (TAC), lipofuscin (LPS), ceruloplasmin (CER), lipid peroxide concentration (LPH) (Table 2).
TABLE 2
Oxidative stress parameters detected in blood and EBC
DETERMINATION OF ACTIVITY OF SUPEROXIDE DISMUTASE (SOD) AND ITS ISOENZYMES
The method of Oyanagui was used to measure the activity of SOD in serum and EBC condensate. In this method, xanthine oxidase produces superoxide anions, which react with hydroxylamine forming nitric ions. These ions react with naphthalene diamine and sulfanilic acid, generating a colored product. Concentration of this product is proportional to the amount of produced superoxide anions and negatively proportional to the activity of SOD. Absorbance was measured using an automated analyzer Perkin Elmer at a wavelength of 550 nm. The enzymatic activity of SOD was expressed in nitric units. The isoenzymes of SOD, such as Mn-SOD and CuZn-SOD, were also identified, using KCN as an inhibitor of CuZn-SOD activity. The activity of SOD is equal to 1 nitric unit (NU) when it inhibits nitric ion production by 50%. Activity of SOD was expressed in NU/ml [15].
DETERMINATION OF PROTEIN SULFHYDRYL GROUPS (SH)
SH concentration was determined in serum and EBC condensate using DTNB, which undergoes reduction by compounds containing sulfhydryl groups, yielding the yellow anion derivative 5-thio-2-nitrobenzoate, which absorbs at a wavelength of 412 nm using a PerkinElmer automated analyzer. The results are shown in μmol/l [16].
DETERMINATION OF CATALASE (CAT) ACTIVITY
Catalase activity in erythrocyte hemolysate and EBC was measured by the method of Johansson and Borg using a PerkinElmer automated analyzer. The method is based on the reaction of the enzyme with methanol in the presence of optimal concentrations of hydrogen peroxide. Formaldehyde produced was measured spectrophotometrically at 550 nm as the dye Purpald. The activity of CAT was expressed as IU/g Hb (hemolysate) or U/l (EBC) [17].
DETERMINATION OF GLUTATHIONE REDUCTASE (GR) ACTIVITY
The activity of GR in erythrocyte hemolysate and EBC condensate was measured according to Richterich using a PerkinElmer automated analyzer. The activity was expressed as IU/g Hb [18].
DETERMINATION OF GLUTATHIONE S-TRANSFERASE (GST) ACTIVITY
The activity of GST in erythrocyte hemolysate and EBC condensate was measured according to the kinetic method of Habig and Jakoby using a PerkinElmer automated analyzer. The activity of GST was expressed as IU/g Hb (hemolysate) or U/l (EBC) [19].
DETERMINATION OF GLUTATHIONE PEROXIDASE (GPX) ACTIVITY
The activity of glutathione peroxidase was measured in erythrocyte hemolysate and EBC condensate by the kinetic method of Paglia and Valentine. The GPx activity was expressed as international units per g of hemoglobin (IU/g Hb) [18].
DETERMINATION OF MALONDIALDEHYDE (MDA)
The product of lipid peroxidation MDA was measured fluorometrically as 2-thiobarbituric acid-reactive substance (TBARS) in serum, erythrocyte hemolysate and EBC condensate according to Ohkawa with modifications. Samples were mixed with 8.1% sodium dodecyl sulfate, 20% acetic acid and 0.8% 2-thiobarbituric acid. After vortexing, samples were incubated for 1 h at 95°C and butanol-pyridine 15 : 1 (v/v) was added. The mixture was shaken for 10 min and then centrifuged. The butanol-pyridine layer was measured fluorometrically at 552 nm and 515 nm excitation (PerkinElmer, USA). TBARS values are expressed as malondialdehyde (MDA) equivalents. Tetraethoxypropane was used as the standard. Concentrations are given in µmol/l of serum/EBC or µmol/g Hb in hemolysate [20].
DETERMINATION OF TOTAL OXIDATION STATUS (TOS)
Total oxidation status was measured according to Erel in serum and EBC condensate. The assay is based on the oxidation of ferrous ion to ferric ion in the presence of various oxidant species in acidic medium. The change in color of the ferric ion by xylenol orange is measured as a change in absorbance at 560 nm. This process is applied to a PerkinElmer automated analyzer and calibrated with hydrogen peroxide. Data are shown in µmol/l [21].
DETERMINATION OF TOTAL ANTIOXIDANT CAPACITY (TAC)
Total antioxidant capacity was measured according to Erel in serum and EBC condensate. In this colorimetric method, radicals are generated, and the antioxidant activity of seminal plasma reduces radical formation. The change in color of ABTS+ ions (2,2′-azinobis(3-ethylbenzothiazoline-6-sulfonate) is measured as the change in absorbance at 660 nm. This method was conducted in a PerkinElmer automated analyzer calibrated with Trolox. Data are shown in mmol/l [22].
DETERMINATION OF LIPOFUSCIN (LPS)
In erythrocyte hemolysate and EBC condensate, the LPS concentrations were determined according to Jain. Fluorescence was measured using an LS45 spectrofluorometer PerkinElmer at wavelengths of 360 nm (absorbance) and 440 nm (emission). Values are presented as relative units (relative fluorescence lipid extract, RF), where X corresponds to a fluorescence solution of 0.1 mg/ml quinidine sulfate in 0.1 N sulfuric acid [23].
DETERMINATION OF CERULOPLASMIN (CER)
The concentration of ceruloplasmin in serum and EBC condensate were determined spectrophotometrically using Richterich reaction with p-phenylenediamine. Ceruloplasmin catalyzes the oxidation of colorless p-phenylenediamine, changing it to a blue-violet dye. PerkinElmer’s VICTORX3 Multilabel Plate Reader with an absorbance filter for 560 nm was used to read samples. Data are shown in md/dl [18].
DETERMINATION OF LIPID PEROXIDE (LPH) CONCENTRATION
In erythrocyte hemolysate and EBC condensate, the LPH concentration was determined according to Södergren et al. using xylen orange. The procedure is based on the oxidation of iron (II) ions to iron (III) ions in an acidic environment. Then the iron (III) ions with xylen orange create a colored complex up to the blue-purple color. The absorbance was measured with a 560 nm filter using a PerkinElmer VICTOR-X3 reader. The concentration was read from the standard curve prepared with the help of appropriate H2O2 concentrations. Values are expressed in μmol /l.
EBC was collected using Ecosreen Turbo condenser (Medivac, Italy). The procedure was performed in the morning, 2 h before meals, and lasted 20 min. The subjects used nose clips and were ordered to periodically swallow saliva to maintain a dry mouth during collection. About 1.5 ml of EBC was collected. Then the EBC samples were stored at –80°C until being analyzed [24].
STATISTICAL ANALYSIS
Results were expressed as median values with interquartile ranges. Nonparametric tests were used (Mann-Whitney U rank sum test and Spearman’s correlation test) because of abnormal distribution of data. All analyses were performed with a software package (Quick Statistica 10). P-values less than 0.05 were considered significant.
RESULTS
Oxidative stress markers have been estimated both in peripheral blood and in EBC among examined patients. However, in comparison to peripheral blood, only a few of those markers were detected in exhaled breath condensate (Table 3). The only detected parameters of oxidative stress in EBC were TAC, TOS, CAT and GST. The values of them were comparable in both groups. Significant differences in the values of oxidative stress parameters detected in patients’ blood between allergic and non-allergic asthma groups were observed with regards to SH, CER, lipofuscin and MDA (Table 3, Figures 1–4). There were no differences in other parameters evaluated in the studied groups.
TABLE 3
Serum and EBC levels of oxidative parameters in asthmatic, allergic and non-allergic patients (values are shown as median and interquartile ranges)
[i] EBC – exhaled breath condensate, SH – sulfhydryl groups, CER – ceruloplasmin, TAC – total anti-oxidant capacity, CAT – catalase activity, GR – glutathione reductase, GPx – glutathione peroxidase, GST – glutathione S-transferase, LPS – lipofuscin, MDA – malondialdehyde, TOS – total oxidation status, LPH – lipid peroxide concentration, SOD – superoxide dismutase activity, MnSOD – manganese superoxide dismutase, CuZnSOD – copper zinc superoxide dismutase.
FIGURE 1
-SH concentration in serum in patients with allergic and non-allergic asthma (square indicates median value, box – interquartile range and whiskers – total range)
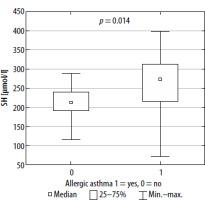
FIGURE 2
CER concentration in serum in patients with allergic and non-allergic asthma (square indicates median value, box – interquartile range and whiskers – total range)
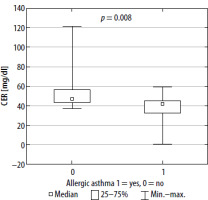
DISCUSSION
EBC is the liquid phase of the exhaled breath samples obtained by cooling. EBC is a non-invasive method for studying the composition of an airway lining fluid [25]. Oxidative stress is involved in various human pathologies such as cardiovascular and neurodegenerative diseases, as well as infections and malignancy [26, 27]. The overwhelming production of ROS and disturbed anti-oxidant activity are critically responsible for the development and sustainment of the inflammatory process. This could be the case in the chronic inflammatory process ongoing in the airways of asthmatics [28, 29]. The results of our study confirmed the role of oxidative stress in asthma pathophysiology and simultaneously the role of inflammation in the complexity of that phenomenon.
Our study indicated that both pro-oxidative stress markers, such as MDA and LPS, and anti-oxidants such as sulfhydryl groups (-SH) and CER, are associated with asthma pathophysiology and allergen sensitization. ROS react with all cell components; however, they cause the greatest damage in proteins, nucleic acids and fats. Since lipid peroxidation is one of the most predominant and recognized consequences of intensified generation of free radicals, oxidative stress evaluation can be based on concentration of MDA, the main product of lipid peroxidation [30]. MDA increases the permeability of membranes for protons and other polar substances, so the difference in electrical potentials of the cell membrane is reduced. Lipid peroxidation also inhibits the activity of some membrane enzymes and transport proteins. These products may also induce the expression of cyclooxygenase-2 (COX-2) in macrophages. Finally, the cell loses the integrity of intracellular membranes and plasma membrane [31, 32]. It has been reported that higher accumulation of lipofuscin may promote generation of ROS, making cells considerably more vulnerable to oxidative stress. It is also known that lipofuscin may act as a danger signal, stimulating the release of proinflammatory chemokines and cytokines, contributing to the activation of macrophages, and therefore leading to a chronic oxidative-inflammatory process [33, 34]. Oxidation of thiol groups (-SH) leads to disturbance of homeostasis of the organism, among other disturbances in the activity of transmembrane proteins, enzymes and transmitters. At the same time, the -SH groups are in equilibrium with glutathione’s thiol groups, whose function is to maintain the thiol groups in the reduced form [35]. Ceruloplasmin is a copper-binding acute-phase protein that increases in inflammatory states. The enzyme is mainly synthesized in the liver but also locally in the brain, retina, hepatocytes, spleen, and lungs. One of the functions of ceruloplasmin is inhibition of metalloproteinase, which is a neutrophil enzyme that promotes oxidative stress in various inflammatory pathologies. Ceruloplasmin also catalyzes oxidation of reactive Fe2+ into Fe3+ (bound to transferrin) [36]. On the other hand, ceruloplasmin may be responsible for LDL oxidation [37].
The search for biomarkers of asthma activity is still a focus of many studies. In spite of the great progress in this field, the clinical relevance of any parameter detected so far is not clearly established. It is equally important, as the current approach to assess asthma control does not include measurements of airway inflammation [29, 38, 39].
It justifies the undertaking of our study aimed at the evaluation of the usefulness of oxidative stress parameters in guiding asthma management. Different biomarkers might not only differentiate the asthma phenotypes/endotypes but also might enable precisely tailored therapeutic intervention, highlighting those patients who remain insufficiently controlled and/or who are at risk of asthma exacerbation. Moreover, the EBC is an easy, non-invasive method of detecting biomarkers, which reflects the inflammation ongoing in the airways. In that context identification of oxidative stress parameters is an attractive observation, but against our expectation we observed that the process is less intense in the airways than in the peripheral blood.
We found only four oxidative stress markers in EBC taken from asthmatics. One can assume the sensitivity of the method used was not sufficient to detect the parameters of that kind of material. In spite of the recommendation of both the American Thoracic Society and the European Respiratory Society Task Force, there is still a lack of standardization of this method [25, 40]. However, the measurement of both the oxidative and anti-oxidative markers in peripheral blood of patients with asthma reflects the inflammatory process in the lungs.
Nevertheless, our results pointed out the role of oxidative stress in asthma pathophysiology. We observed a higher level of oxidative stress markers and lower values of anti-oxidative parameters in blood in non-allergic asthma patients in comparison to allergic patients. The obtained results provide evidence supporting a role for a more intense inflammatory process in non-allergic asthma.
Although the results of our study confirm the role of oxidative stress in asthma pathophysiology, against our expectation the clinical relevance of the parameters detected is still not proved. None of them are useful enough in guiding asthma management decisions. One can assume that ROS is the reasonable goal of the future therapeutic strategy aimed at alleviation of oxidative stress-induced inflammation. The redox mechanism presents a promising therapeutic target in asthma. Both the decreased exposure to environmental reactive oxygen species and augmentation of the endogenous antioxidant defenses might bring benefit in asthma management. ROS are responsible for many pathophysiologic elements of the inflammatory process. In that context, it is worth noting the role of the oxidized cysteine redox state in inhibition of glucocorticosteroid receptor function. It is equally important, as glucocorticosteroids are the mainstay of asthma management [41, 42]. A therapeutic intervention that decreases the oxidative stress in the airways and augments endogenous antioxidant defenses might be beneficial as an adjunctive therapy in asthmatic patients.
CONCLUSIONS
Both the oxidant activity and antioxidant capacity are detected in peripheral blood level and in an exhaled breath condensate. The inflammatory process is increased in non-allergic asthma in comparison to allergic asthma. Exhaled breath condensate is only partially useful to assess airway inflammation.