Introduction
Over the last year, the incidence of melanoma worldwide has been increasing faster than other cancers around the globe [1]. The epidemiological assessment estimated that 325 000 new cases and 57 000 deaths occurred in 2020. The global burden is forecast to reach 510 000 new cases and 96 000 deaths by 2040 [2]. Australia and New Zealand have currently the highest incidence for males (42 per 100 000 person-years) and females (31 per 100 000 person-years) [2]. Therefore, it represents an important health burden. Patients with metastatic melanoma currently have few therapy options. Therefore, to create effective and improved therapies, it is critical to investigate the genetic and epigenetic causes of melanoma. Regarding the alterations in protein-coding genes, especially small non-coding RNAs, microRNAs are considered. In recent years, miRNA-based therapy has garnered significant attention for its effectiveness in cancer treatment.
MiRNAs, small non-coding RNAs, play crucial roles as regulators of cellular processes including differentiation, proliferation, metastasis, and apoptosis. They facilitate the transportation of diverse payloads, such as proteins, miRNA, mRNA, lipids, and complex carbohydrates, resulting in a range of particular cellular functions. Tumors often exhibit dysregulation of these factors. They act as oncogenes or tumor suppressors [3]. Oncogenes play a role in promoting the development of cancer by negatively regulating tumor suppressor genes, as well as genes responsible for controlling cell differentiation or apoptosis. By modulating oncogenes and genes involved in apoptosis or cell differentiation regulation, tumor suppressor genes limit cancer [4]. Several known human miRNAs demonstrate dysregulated expression levels, some of which increased and some of which decreased in melanoma [4].
One of the tumor-suppressor miRNAs is miRNA-211a. Several studies have reported the effect of miRNA-211a on various transcription factors and its essential function in melanoma. Its low expression in melanoma was reported [5]. Decreased levels of miR-211 in melanoma via post-transcriptional regulation of target mRNAs would lead to tumor progression and tumorigenesis [6]. Therefore, increasing the level of miRNA-211a in melanoma cells by effects on target genes can prevent the growth of cancer cells.
Among the various miRNA-211 targets are TGFBR2, EZRIN (a cytoskeleton- associated protein, and a member of the ezrin-radixin-moesin family), and nuclear factor of activated T-cells 5. miRNA-211a has important roles in signaling pathways, including melanocyte inducing transcription factor (MITF)/brain-specific homeobox (BRN2), and potassium calcium-activated channel subfamily Mα1 (KCNMA1)/transient receptor potential cation channel subfamily M member 1 (TRPM1)/MITF [5]. High expression of miR-211 via targeting BRN2 and KCNMA1 inhibits the invasive and metastatic ability of melanoma cell lines [7]. Furthermore, miRNA-211, which affects BRN2, causes cell death or prevents the formation of melanoma tumors [8]. Furthermore, miR-211 targets transforming growth factor-β receptor (TGFBR2) and vascular endothelial growth factor (VEGF). It prevents the growth and migration of tumor cells in this manner [9, 10].
One of the principal obstacles to suitable application of miRNAs for the treatment of various cancers is the absence of an effective nano-vehicle. In recent years, exosomes were introduced and used as a fantastic nano-carrier for carrying such nucleic acids as miRNAs in therapeutic approaches [11]. These natural nanoparticles with sizes in the range 30–150 nm are released from all kinds of cells [12]. Exosomes encompass nucleic acids, proteins, lipids, cytokines, transcription factor receptors, and various other bioactive compounds [13]. Exosomes may carry a wide range of bioactive chemicals, including those that break down quickly, to specific cells to engage in regulatory functions such as immune regulation, tumor development and therapy, tissue repair, and tumor identification [13, 14].
Some advantages of exosomes as therapeutic carriers are homogeneity, low immunogenicity, and low toxicity [15]. Exosomes are more readily produced, stable in bodily fluids, biocompatible, and biodegradable. Their tiny size allows them to quickly evade clearance, cross the blood-brain barrier and be internalized by way of fusion with the plasma membrane [16]. TEXs contain tumor-associated and specific antigens, and a lot of natural microRNAs that thereby affect the immune system [17].
Based on a number of additional studies, tumor-derived exosomes enriched by miRNA (TEXomiR) as suitable carriers accelerated the death of malignant cells [18–20].
Therefore, these findings and characteristics make engineered exosomes suitable candidates for therapeutic goals. Considering the global incidence of melanoma, and the failure of current treatment methods, the goal of the present study was to evaluate the efficiency of encapsulated exosomes with miR-211a and their effects on proliferation, apoptosis, and expression of B16F10 cells’ target genes.
Material and methods
Cell line
B16F10 murine melanoma cells were obtained from Pasture Institute, Iran. The cells were grown in Dulbecco’s Modified Eagle’s Medium (DMEM, Sigma-Aldrich, USA) High Glucose according to the instructions provided by ATCC. DMEM media contained 10% fetal bovine serum (FBS) (Gibco, Germany) and 100 U/ml penicillin/streptomycin (Gibco, Germany). The B16F10 cells were incubated in 5% CO2 at 37°C. Once the density of the cell growth reached approximately 75%, the cells were trypsinized.
Preparation of exosomes
To eliminate FBS exosomes and the inhibition of their interference with TEXs, the B16F10 cells were adapted to an FBS-free condition based on the following methods [18, 21]. The adaptation of cells to serum-free conditions was initiated with cells at a density of 2.5 × 105 cells per well and viability of more than 85% at a 10% FBS. The fetal bovine serum percentage gradually decreased 10–0% daily in the culture medium. In the last stage, once the cells were entirely adapted in FBS-free DMEM, the cells were incubated at 37°C for 72 hours. Afterward, supernatants were gathered for extraction of exosomes using an Exocib kit (Cibzist, Tehran, Iran, Catalog number: 3603-450). In brief, supernatants were centrifuged for 10 min at 350 g and passed through a filter with a 0.22 µm pore size. Then reagent A of the Exocib kit was added to supernatants at a ratio of 1 : 5, respectively, and vortexed for 5 min. The mixture was stored overnight at 4°C and was shaken every one hour. Next, the sample was centrifuged at 350 g for 45 min. Subsequently, the supernatant was removed and the pellet mixed with 150 µl of cold particle-free phosphate-buffered saline (PBS). Isolated exosomes were cryopreserved at –80°C.
Characterization of exosomes
A bicinchoninic acid (BCA) protein assay kit (DNAbioTech, Tehran, Iran) was used to quantify the concentration of exosome proteins. In brief, the standards, consisting of six different concentrations (range 0–1000 µg/ml) of bovine serum albumin protein (BSA, powder) (Sigma-Aldrich, USA, catalog number: A2058), were prepared and diluted based on the manufacturer’s instructions. To each microtube, 10 µl of exosomes and standards were added, followed by 10 µl of the working solution. After thorough shaking, the microtubes were incubated at 55°C for 25 minutes. After cooling, absorbance at 56 nm was measured using a NanoDrop instrument (NanoDrop 2000, USA). Transmission electron microscopy (TEM) (Philips CM30 electron microscope, Eindhoven, Netherlands) and scanning electron microscopy (SEM) (TESCAN-Vega3, Czech Republic) were used to image the morphological shape of the exosome. In short, 1 µg/µl of purified exosomes were fixed with 2% paraformaldehyde for 1 hour. The fixed exosomes were mounted on copper grids. Finally, the grids are allowed to air dry for a duration of 30 minutes. Next, exosomes were stained and treated with a 1% uranyl acetate solution to provide contrast for negative staining. The isolated exosomes were dried on a glass substrate for 24 hours at a concentration of 1 µg/ml in order to prepare them for SEM imaging. Then, a coating of 2–5 nm gold was applied by sputtering and then directly examined using an SEM instrument. Dynamic light scattering (DLS) was used to evaluate the distribution of exosome size. A suspension of exosomes and PBS at a ratio of 1 : 1000, respectively, was diluted and mixed. The mixture was injected into the laser chamber of a Nano Zeta sizer (Malvern Instruments Ltd., Malvern, UK). Experiments were carried out in triplicate and then analyzed. The Western blot method was used to determine the expression of exosome markers, including CD9, CD63 and CD81. The exosomes were treated with RIPA buffer from a radioimmune precipitation assay kit (Sigma-Aldrich, Canada) to extract total protein. SDS-PAGE was then used to separate this protein at 12.5%. The proteins were then transferred to a nitrocellulose membrane and treated overnight with mouse anti-CD9, CD63, and CD81 antibodies (Santa Cruz Biotechnology, TX, USA). Afterward, bound antibodies were detected by incubating with horseradish peroxidase-conjugated secondary antibody (Sigma- Aldrich, Canada) for one hour. All experiments were run in triplicate.
Loading of miR-211a mimic in exosomes
The calcium chloride method, a transfection method introduced by Zhang et al. [22], was used for exosome loading with miR-211a mimic (Bon Yakhteh, Tehran, Iran). A 1 : 1 solution of 2 µg/µl of exosome, 200 pmol miR-211a mimic, and CaCl2 solution 0.1 M with the final volume set to 300 µl was mixed. The suspension was placed on ice for 30 min, followed by incubation at 42°C for 60 s, and finally returned to ice for 5 min. To eliminate free-floating miRNA-211a mimics outside the exosomes, the mixture was incubated with RNase A (Thermo Fisher, Scientific, Waltham, USA). Subsequently, for inactivation of RNase A, the mixture was treated with 5 µg/ml RNase inhibitor (Yekta Tajhiz Azma, Tehran, Iran) for 15 min at 37°C.
TEXomiR were re-isolated using an Exocib kit. Encapsulation efficiency of miRNA-211a was proved by stem-loop real-time polymerase chain reaction (PCR) assays.
The following formula was applied to evaluate the effectiveness of encapsulation [20]:
Vc is exogenous control volume; Cc is exogenous control concentration; MWc refers to the molecular weight of exogenous control; REs is the relative expression of miRNA-211a mimic; Vs is the volume of transfected miRNA; Cs is transfected miRNA concentration; MWs is the molecular weight of transfected miRNA; REc is control relative expression.
Cell viability assay
MTT (3-(4,5-dimethylthiazol-2-yl)-2,5-diphenyl tetrazolium bromide) (1 mg/ml, Sigma, USA) assay was conducted to determine the cell viability based on the manufacturer’s protocol (Kiazist, Iran). Concisely, a solution containing 10 ∧4 cells/well of B16F10 cell lines was evenly dispersed in 96-well plates prior to overnight treatment. The cells were exposed to five different concentrations (5, 10, 25, 50, and 100 µg/ml) of TEX and TEXomiR over 24 and 48 hours. Then, 10 µl of MTT was added to the medium in each well and incubated for 4 h at 37°C. Afterward, the supernatant was removed. Then, 200 µl of dimethylsulfoxide (Sigma, USA) was added. After 10 minutes, optical density values were calculated at 540 nm (Denovix, Wilmington, DE, USA) on a microplate reader. The findings were read in triplicate.
RNA isolation and real-time polymerase chain reaction
To assess the effectiveness of delivering TEXomiR in a laboratory setting, a total of 100,000 B16F10 cell lines were placed in each well of a 48-well plate. The cells were then exposed to either exosomes alone or exosomes loaded with miRNA-211a for 48 hours. Subsequently, the levels of miR-211 and target genes were assessed using real-time PCR. For this, total RNA was isolated using RNX Plus (Sina Clone Co., Tehran, Iran, Catalog number; EX6101) following the manufacturer’s protocol. The quality of isolated RNA was evaluated by a Nanodrop 2000C (Thermo Scientific, USA). Isolated RNA was stored at –80°C. To analyze miRNA, cDNA was generated using a poly (A)-based miRNA cDNA synthesis kit (Bon Yakhteh, Tehran, Iran). Afterward, the expression of miRNA-211a was conducted using a SYBR Green gene expression Master Mix kit (Ampliqon, Odense, Denmark) and specific primers procured from Bon Yakhteh (Tehran, Iran). For mRNA analysis, cDNA was synthesized using a cDNA synthesis kit (Yekta Tajhiz, Tehran, Iran). Subsequently, expression levels of miRNA-211a, BRN2 (ID: 18992), VEGF (ID: 22339), and TGFBR2 (ID: 21813) genes (Sinaclon, Iran) were measured by the Applied Biosystems StepOne Real-Time PCR Systems. The amount of miRNA-211a and target genes were normalized by an endogenous control U48 snRNA and gapdh gene, respectively. Quantification levels was calculated with the 2ΔΔCt method. All experiments were performed in triplicate. The specific primers for miRNA-211a and U48 snRNA were procured from Bon Yakhteh (Tehran, Iran). Primers were designed and checked using the NCBI Primer-Blast Tool (NCBI database):
VEGF forward primer: TCGCTCCTCCACTTCTGAGG,
VEGF reverse primer: GGCCATTACCAGGCCTCTTC;
TGFBR2 forward primer: GACCAGAAATTCCCAGCTTCT,
TGFBR2 reverse primer: CAACGTCTCACACACCATCTG;
Brn-2 forward primer: CGGCGGTTTGCTCTATTC,
Brn-2 reverse primer: ATGGTGTGGCTCATCGTG,
gapdh forward primer: AGGTCGGTGTGAACGGATTTG,
gapdh reverse primer: TGTAGACCATGTAGTTGAGGTCA.
Apoptosis assay
1 × 105 B16F10 cells were allocated to each well of a 24-well plate in the control, unloaded TEX and TEXomiR groups, and incubated overnight. Then 25 µg/ml of TEX and loaded TEX were added to the related wells. After 48 hours, the treated cells were gently trypsinized and washed with PBS. Then, 2 µl of Annexin V-FITC (BioLegend, San Diego, USA) and 5 µl of PI (propidium iodide) (BioLegend, San Diego, USA) were added to each experimental group cell. Following a 5–15-minute incubation period at room temperature in the absence of light, the tubes containing the cells were rinsed twice with PBS. Upon the addition of 400 µl of PBS, the specimens underwent analysis utilizing a Becton Dickinson FACScan system.
Statistical analysis
Data were analyzed as mean ±SD. One-way analysis of variation (ANOVA) was used in this study to evaluate the relationship among different groups. The level of statistical significance was defined as p < 0.05. The results were then analyzed by GraphPad Prism 5 (GraphPad Software Inc., La Jolla, CA, USA).
Results
Characterization of exosomes
The results showed that B16F10 cells adapted to FBS-free condition have no significant morphological changes (Fig. 1 A). The total protein concentration of the exosome, as determined by the BCA assay, was 3600 µg/150 ml. The size of exosomes derived from B16F10 cells was determined to be between 30 and 130 nanometers, as confirmed by TEM (Fig. 1 B) and scanning electron microscope (Fig. 1 C) analyses. Moreover, based on DLS results, the average exosome size was 90 nm (Fig. 1 D). The Western blotting results validated the presence of CD9, CD63, and CD81 in isolated exosomes (Fig. 1 E).
Fig. 1
Characterization of B16F10 cell isolated exosomes. Light microscope image of B16F10 cells’ morphology. Scale bar: 50 μm (A), transmission electron microscopic image of exosomes derived from B16F10-tumor-derived exosomes (TEXs) (B), image taken of B16F10-derived TEXs using a scanning electron microscope (C), dynamic light scattering results showing the size distribution of B16F10-derived TEXs (D), western blot results, TEX, tumor exosome (E)

Efficient delivery of miR-211
The results showed that miRNA loading efficiency was 43%; p < 0.01. Compared with the unloaded TEX group, the relative gene expression levels of miR-211 in the TEXomiR group were significantly higher (relative expression: 0.7 ±0.2 vs. 14.3 ±0.6) (Fig. 2 A). These results confirm the successful overexpression of miR-211 in B16F10 cells that were treated with TEXomiR (Fig. 2 B). Relative expression levels of miR-211 in each group of control, TEX, and TEXomiR were 0.4 ±0.1, 3.7 ±1.6, and 21 ±5.6 respectively (p < 0.0001).
Fig. 2
Efficient encapsulation of exosomes by miR-211. Expression level of miR-211 mimic in encapsulated exosome was measured using real- time polymerase chain reaction (A), relative expression of miR-211 in B16F10 cell treated by tumor-derived exosomes (TEX)omiR or TEXs (B)
The results are reported as mean ±SD.
**** p < 0.0001
PBS – phosphate-buffered saline, TEX – tumor-derived exosomes, TEXomiR – tumor-derived exosomes enriched by miRNA
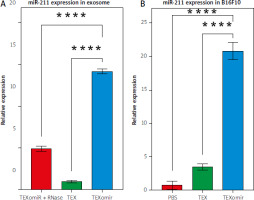
Cytotoxic effect of TEXomiR on B16F10 cells
To study the cytotoxicity of exosomes against B16F10 cells, the effect of TEX and TEXomiR on B16F10 cells was evaluated by MTT assay. After 24 hours, the administration of TEX at various dosages had a discernible effect on the survival rate of B16F10 cells, in comparison to the control group. The cell viability % of TEXomiR-treated groups showed a significant drop compared to the controls, as shown by statistical analysis (p < 0.01) (Fig. 3 A, B). The viability of B16F10 cells treated with TEXomiR decreased with an increasing concentration compared with the control group after 48 hours. The results showed a dramatic reduction after treatment with 25 µg/ml of TEXomiR compared with the control group (Fig. 3 B).
Fig. 3
B16F10 cell viability. B16F10 cells with various concentrations (5, 10, 25, 50, and 100 μg/ml) of tumor-derived exosomes and tumor-derived exosomes enriched with miRNA (TEXomiR) compared to the control group after overnight treatment (A), TEXomiR showed significant cytotoxicity following treatment with 25 μg/ml after 48 hours (B)
The data are obtained from three experiments and presented as mean ±SD (*** p < 0.001, ** p < 0.01) vs. control group.
TEX – tumor-derived exosomes, TEXomiR – tumor-derived exosomes enriched by miRNA
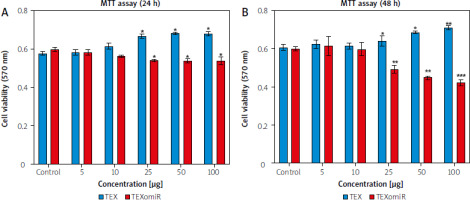
Effects of TEXomiR on expression level of target genes
The expression of VEGF, TGFBR2, and BRN2 mRNA showed that following treatment of B16F10 cells with TEXomiR, mRNA levels of VEGF (Fig. 4 A) and TGFBR2 (Fig. 4 B) were significantly downregulated in the TEXomiR group compared with TEX and control groups (relative expression = 0.49 ±0.16, p < 0.01 and 0.57 ±0.17, p < 0.01, respectively). Although the expression of BRN2 mRNA was lower than in the control group, the difference was not significant (Fig. 4 C). These data showed that TEXomiR was taken up and internalized by B16F10 cells successfully.
Fig. 4
Relative expression levels of vascular endothelial growth factor (A), transforming growht factor, β-receptor II (B), and brain-specific homeobox were detected (C)
Results are reported as mean ±SD.
** p < 0.01
PBS – phosphate-buffered saline, TEX – tumor-derived exosomes, TEXomiR – tumor-derived exosomes enriched with miRNA
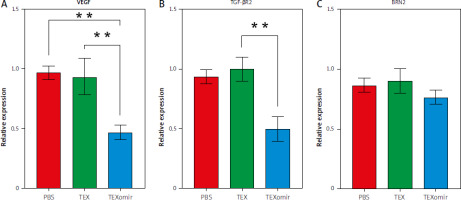
miRNA-enriched exosomes induced apoptosis in B16F10 cells
Flow cytometry was used to identify the apoptosis of B16F10 cells treated with TEX and TEXomiR (25 µg/ml) after 48 hours (Fig. 5). Based on the results obtained, apoptotic effects of TEXomiR on B16F10 cells resulted in a higher rate of apoptosis compared with TEX (p < 0.001) and control (p < 0.001) groups (apoptosis %: TEX = 27.71, TEXomiR = 40.7, control = 5.29). Moreover, the apoptotic effect was not only observed in the TEXomiR group. The TEX alone group showed a higher rate of apoptosis than in the untreated cells.
Discussion
miRNAs play a significant role in cancer, and miRNA- based therapies have been a focal point of attention for numerous years [23]. Exosomes, which are very effective carriers, may transport and protect contained miRNAs from enzymatic degradation, while delivering them specifically to target cells with little adverse consequences. This ensures that destination cells get the highest possible quantities of miRNAs [24, 25]. Since we were unable to achieve successful loading efficiency via electroporation, we turned to a modified calcium chloride method for delivering miRNA. In this research, we innovatively introduced miR-211 into exosomes through calcium chloride-mediated transfection to optimize their suitability for miRNA delivery. Moreover, calcium chloride has the ability to aid in loading miRNAs onto exosomes, thus decreasing the necessity for specialized laboratory tools. Based on cell origin, exosome purity, and treatment duration, our findings are successful, and align with other research. Cheng et al. described transfection of miR-125a/b mimic into exosomes derived from jejunum tissue of rats by calcium chloride-mediated transfection [26]. Rezaei et al. reported that tumor-derived exosomes encapsulated by miRNA-124 promoted an anti-tumor immune response in CT-26 tumor-bearing mice. They applied a modified calcium chloride method to deliver miR-mimic into the exosomes, achieving the efficiency of 53.2% [20]. The results of our study demonstrated that the expression of miR-211 encapsulated in TEX was significantly higher by 43-fold when compared to exosomes alone. The findings presented here align with previous research that demonstrated the 42.5-fold transfer of miR-34a mimetic to CT-26 via calcium chloride transfection [21], which was carried out by exosomes. Also, Zhang et al. reported delivery of miR-15a mimic enriched with exosomes to THP-1 macrophages via the calcium chloride method [22].
In the next step the study results indicated that exposing the B16F10 cell line to exosomes enriched with miRNA-211a effectively inhibits proliferation of the B16F10 cell line, induces apoptosis, and suppresses cancer cell metastasis. We observed that miR-211 mimics prevent the proliferation of cancer cells. This is achieved via the regulation of a crucial set of genes associated with cancer.
Multiple studies have reported, consistently with our observations, that exosomes promote the proliferation of cancer cells on their own. In a study, Sun et al. [27] established that the administration of isolated exosomes or miR-21 to colon cancer cells led to the upregulation of genes that are linked to cellular invasion and proliferation. Bo Wang reported that in both in vitro and in vivo settings, exosome miR-1910-3p enhanced the proliferation and migration of breast cancer cells [28]. In contrast with our study, some studies have proven the anti-proliferation effects of exosomes on tumor cells [18, 29, 30]. Apoptosis of CD4+ T-cells was demonstrated to be induced by exosomes derived from B16 through the downregulation of anti-apoptotic proteins (BCL-2, MCL-1, and BCL-xL) and the activation of caspase-3, caspase-7, and caspase-9 [31]. Moreover, Shen et al. reported that pancreatic cancer- derived exosomes induced apoptosis of T lymphocytes via the p38 MAPK-mediated endoplasmic reticulum stress [32].
Our findings demonstrate that the incorporation of miR-211 into exosomes induces apoptotic effects. Thus, after 48 hours of treatment, TEXomiR induced a progressive increase in apoptosis of B16F10 cells.
Previous studies have examined the effect of increased miRNA expression in cell lines. It was reported that the upregulation of miR-211 mimics could prevent the proliferation of various cancer lines such as thyroid cancer cell lines [33], HeLa, C33A, SiHa, and CaSki cervical cancer cell lines [34], MDA-MB-231 and MCF-7 breast cancer cell lines [35] and G361, GR-M and OCM-1 melanoma cell lines [36].
In some studies, the researchers transfected cell lines by mimicking miRNAs and investigated the effects of released exosomes on apoptosis [37, 38]. By increasing the expression of Bax, exosomal miR-22 upregulated apoptotic pathways in cervical cancer cells, according to a study by Konishi et al. [39]. Surui Yao et al. examined the consequences of exosome transduction by lentivirus. The results demonstrated that exosomal miR-204-5p could induce apoptosis in HEK293 T-cell line [40].
While the outcomes may vary based on the cell origin, exosome dosage, and treatment duration, our findings indicate that transfecting exosomes with miR-211 can trigger dose-dependent apoptosis in B16F10 cells.
According to our findings, exosomes alone may cause B16F10 cells to undergo apoptosis, although not as much as they can when combined with TEXomiR. It is hypothesized that under conditions of cellular starvation, cells may generate and release autophagosomal vesicles and exosomes for removing toxic compounds from the cells [41, 42].
Therefore, when tumor cells are treated with TEX generated from starved cells, the result is an apoptotic tumor as opposed to isolated exosomes under normal circumstances. Further research is needed to explore the specific signaling pathways responsible for the tumor-suppressing effects of starved texosomes.
One of the target genes of miR-211 is BRN2 transcription factor, which has a vital role in apoptotic pathways [8]. The results of our study showed that although the mRNA expression level of BRN2 was downregulated after treatment of B16F10 by miR-211-enriched TEX, it was not statistically significantly lower than in the control group. Several studies have found that the upregulation of miR-211 results in downregulation of BRN2 and induces apoptosis in tumor cells [43]. In contrast, Ray et al. highlighted the paradoxical roles of mir-211 in melanoma cells. They stated that the initial stage of miR-211 or BRN2 can influence cell fate. So, elevated BRN2/low miR-211 leads to tumor progression, high BRN2/high miR-211 results in tumor suppression, and high BRN2/intermediate miR-211 along with other factors can lead in either direction [8].
Our results indicated that TEX enriched with miR-211 significantly decreased the expression of VEGF in comparison to the control or exosome-treated group. In line with our observations, Xin et al. reported that overexpression of miR-211 suppressed the proliferation and migratory capacities of cutaneous melanoma cells. Their study demonstrated that the excessive production of miR-211 effectively reduced the expression of VEGF [44]. Moreover, it was reported that upregulation of miR-211-5p reduced the proliferation, migration, and angiogenesis of hepatocellular carcinoma cells. Also elevated miR-211-5p decreased the expression of VEGF-A [10].
We observed decreased expression levels of TGF-βR2 after treatment of B16F10 cells with miR-211-enriched TEX. Consistent with our research, Levy et al. reported that reduced levels of TGF-βR2 following up-regulation of miR-211 led to a decrease in the migration and invasion of melanoma cells [45]. Dai et al. reported that miR-211 is a reverse regulator of TGF-β signaling so delivery of pre-miR-211 into melb-a cells resulted in a decrease in TGF-βR2 mRNA levels. The study demonstrated the significant involvement of miR-211 in pigmentation and the preservation of melanocyte stem cells in living organisms via its impact on TGF-β signaling [9].
Even though our study showed an apoptotic and cytotoxic effect, there are some limitations of the study, as follows: 1) Although B16F10 cells were adapted to a culture medium without FBS and they did not show any phenotypical and morphological effects, this circumstance may affect the quality and quantity of produced exosomes; 2) The loading efficiency of our work was moderate because we used CaCL2 loading buffer for transfection of miR-211 mimic into the exosome instead of cloning by electroporation.
Among the obstacles to the therapeutic use of nanoparticles are the source of the cells, the low exosome separation efficiency, and the lack of targeted exosomes. We demonstrated the capacity of exosomes not only as carriers but also as effective inhibitors of cancer growth.
Conclusions
The findings of our study indicate that exosomes have the capability to transport miR-211 to B16F10 cells. Our research indicates that miR-211-enriched TEX, as a natural therapeutic method, may trigger apoptosis and reduce viability in melanoma cell lines. More studies are required to clarify the therapeutic effect of TEXomiR in in vivo model systems.