Introduction
Gastric cancer is the cancer type with the fifth highest incidence and fourth highest mortality, resulting in more than 1 million new cases and more than 750,000 deaths every year [1]. In 2020, there were more than 5,000 new cases in female patients and more than 9,000 new cases in male patients in Germany [2]. The relative five-year survival rate is only 37% in women and 35% in men [2]. Risk factors to develop a gastric tumor are mani-fold and include, among others, Helicobacter pylori infection, gastroesopha-geal reflux, high salt intake, being male, and consuming alcohol and red meat [3, 4]. Both mortality and morbidity of gastric cancer are declining, probably due to better hygiene, a reduction in Helicobacter pylori infections and improvements in terms of therapies [5, 6]. Current treatment options include endoscopic resection, surgery with lymphadenectomy and different chemotherapy regimens [7]. Gastric tumors are infiltrated with immune cells, which can be used not only to identify different molecular subtypes, but also to predict the response to immune checkpoint inhibitors [8].
Interleukin-6 (IL-6) and IL-11 both belong to the IL-6 family of cytokines [9] and have partly overlapping, but mostly unique, biological functions [10]. Both cytokines can be secreted by a variety of different cell types, including different types of immune cells, epithelial and endothelial cells and fibroblasts [10, 11]. A typical inducer of IL-6 production is lipopolysaccharide derived from gram-negative bacteria [12], while IL-11 is induced e.g. by transforming growth factor (TGF-β1) or infection with respiratory syncytial virus [13]. IL-6 and IL-11 activate their target cells via binding to non-signaling α-receptors at the cell surface (IL-6R and IL-11R, respectively). The resulting IL-6/ IL-6R and IL-11/IL-11R complexes induce the formation of a homodimer of the signal-transducing β-receptor gp130, which in turn activates intracellular signaling cascades, most notably the Janus kinase/signal transducer and activator of transcription (Jak/STAT) pathway [9, 14]. Here, Jak1 phosphorylates tyrosine residues within the intracellular part of gp130, which serve as docking sites for STAT3, which are then also phosphorylated and translocate into the nucleus to induce target gene transcription [14, 15]. While gp130 is ubiquitously expressed, IL-6R and IL-11R expression are restricted to certain cell types, and thus the expression pattern of IL-6R and IL-11R dictates which cell type responds to which cytokine. However, soluble forms of both IL-6R and IL-11R (sIL-6R/sIL-11R) exist, which are generated by proteolytic cleavage of the membrane-bound receptors and are able to bind their ligand with the same affinity [16–20]. Signaling via soluble receptors is called trans- signaling and accounts in the case of IL-6 for the pro-inflammatory properties of the cytokine, while this is not known for IL-11 yet [21–23].
Serum levels of both IL-6 and IL-11 are very low or even undetectable in healthy humans, but can rise substantially during disease, which is exemplified by IL-6 levels of more than 100 ng/ml in patients with meningococcal septic shock [24]. In most inflammatory diseases, however, serum levels of IL-6 and IL-11 are in the pg/ml or low ng/ml range. The involvement of IL-6 in most inflammatory diseases made the cytokine and its receptor an attractive therapeutic target, which resulted in the development and clinical admission of several antibodies blocking either IL-6 or the IL-6R [25, 26]. Selective blockade of IL-6 trans-signaling, which appears to be superior compared to global blockade of IL-6 [27], is currently being evaluated in patients with inflammatory bowel disease [28, 29] and atherosclerotic cardiovascular disease [30]. Antibodies targeting IL-11 or the IL-11R are currently under development, as IL-11 appears to be a druggable target in different fibrotic diseases [31, 32].
Chronic inflammation is closely correlated to gastric tumorigenesis, and cytokines of the IL-6 family play crucial roles in both processes [33, 34]. Helicobacter pylori infection increases IL-6 expression in patients and both IL-6 and IL-11 have been reported to be strongly up-regulated in biopsies of gastric cancer patients [35]. IL-11 has also been identified by proteomics in human gastric tumor samples [36]. In addition, several mechanistic studies have investigated the roles of IL-6, IL-11, gp130 and STAT3 using rodent models with genetically modified mice. The gp130757F/F-mice, in which the tyrosine residue 757 of gp130 is replaced by a phenylalanine residue, resulting in loss of negative feedback and thus overactivation of gp130-mediated STAT3 signaling, develops gastric tumors spontaneously [37]. The major driver in this model is IL-11, as genetic deletion of the IL-11R prevents gastric tumor formation, while deletion of IL-6 has little to no impact [38–41].
In the present study, we analyzed expression of IL-6R, IL-11R and gp130 in three gastric tumor cell lines and three monocytic immune cell lines. Using recombinant proteins, we analyzed the response of the different cell lines to stimulation with the different cytokines. Furthermore, we used in silico analyses to evaluate the prognostic value of the expression of the different genes in terms of overall survival of gastric cancer patients.
Material and methods
In silico analyses
All in silico analyses were performed using GEPIA2 (http://gepia2.cancer-pku.cn/) [42]. Data were retrieved and analyses performed on December 6th, 2023. For this study, we used the stomach adenocarcinoma (STAD) dataset, consisting of 408 tumor samples, 36 normal controls, and 175 GTEx samples (stomach). We generated survival plots using the “survival analysis” package using standard parameters except that we did not include the 95% confidence interval. We used the “Expression DIY” package to generate the box plots for the expression analysis of the different genes in tumors compared to normal and GTEx data using standard parameters.
Reagents
The following primary antibodies were used: β-actin (D6A8), GAPDH (D16H11) XP® Rabbit mAb, phospho-STAT3 (Tyr705, D3A7) XP® Rabbit mAb and STAT3 (124H6) Mouse mAb (all from Cell Signaling Technology, Danvers, MA, USA). Secondary antibodies were anti-mouse IgG, HRP-linked anti-body and anti-rabbit IgG, HRP-linked antibody (both also from Cell Signaling Technology). Hyper-IL-6, IL-6 and IL-11 were produced in house as described previously [43–45].
Cell lines and cell culture
CLS-103, NCI-N87, THP-1 and U-937 cells were cultivated in RPMI 1640 medium (PAN-Biotech or Thermo Fisher Scientific). J774A.1 cells were cultured in DMEM medium and AGS cells in Ham’s medium (both from PAN-Biotech, Aidenbach, Germany). All media were supplemented with 10% fetal bovine serum (Thermo Fisher Scientific, Waltham, USA), 100 U/ml penicillin and 100 µg/ml streptomycin (PAN-Biotech, Aidenbach, Germany). Ham’s medium was additionally supplemented with 200 mM L-glutamine (PAN-Biotech, Aidenbach, Germany). All cells were kept at 37°C and 5% CO2 in a standard incubator with a water- saturated atmosphere.
Stimulation of cell lines with cytokines
In order to analyze STAT3 signaling in the different cell lines, the following cell numbers of adherent cells were seeded in 6-well plates in 2 ml medium per well: 5 × 105 AGS cells, 5 × 105 CLS-103 cells and 1 × 106 NCI-N87 cells. The cells were placed in the incubator overnight and the medium was removed the next day. After three washing steps with 1 ml PBS each, the cells were serum-starved for 24 hours in 1 ml of medium without serum. The suspension cell lines were collected by centrifugation, the cells washed three times with 10 ml of PBS each, and then re-suspended in serum-free medium. The following cell numbers were seeded in 12-well plates in 1 ml of medium per well: 3 × 106 J774.A1 cells, 1.5 × 106 THP-1 cells and 1.5 × 106 U-937 cells. The monocyte cell lines were serum starved for 6 hours. After incubation, cytokines were added to the medium at the following concentrations: 10 ng/ml IL-6, 10 ng/ml IL-11 and 10 ng/ml Hyper-IL-6 (HyIL-6). For THP-1 and U-937 cells, 50 ng/ml Hyper-IL-6 was used instead. The cells were placed back into the incubator for 15 min. The cells were detached and washed in PBS and finally lysed in lysis buffer (10 mM Tris-HCl, 140 mM NaCl, 5 mM EDTA, 1% Triton-X 100, protease inhibitor cocktail and phosphatase inhibitor cocktail (both Sigma-Aldrich), pH 7.5).
Western blotting
Cell lysates were separated by SDS-PAGE and transferred to nitrocellulose membranes. Membranes were blocked in tris-buffered saline (TBS) supplemented with 5% albumin fraction V and 0.2% Nonidet P40 for 1 h at room temperature. Afterwards, the membranes were incubated with the primary antibodies (diluted 1 : 1,000 in TBS-T [TBS supplemented with 0.25% Tween-20] with 5% bovine serum albumin) at 4°C overnight. After washing three times with TBS-T, the membranes were incubated with secondary antibo-dies (diluted 1 : 3,000 in TBS-T supplemented with 5% milk powder) for 1 hour at room temperature. Membranes were again washed with TBS-T and afterwards incubated with Immobilon Western HRP substrate (Millipore by Merck, Darmstadt, Germany) and detected using the Western Blot Imager FluorChem E (Biosciences, Santa Clara, USA). For detection of total STAT3 and the loading control GAPDH after detection of pSTAT3, membranes were stripped, washed with TBS-T, and again blocked and incubated with antibodies as described above.
Densitometric analysis
To quantify pSTAT3 levels, western blot images were densitometrically analyzed using the Image Studio Lite software (LI-COR Biosciences) and the ratio of the pSTAT3/STAT3 signal intensities was calculated in relation to the unstimulated control.
RNA isolation and quantitative real time polymerase chain reaction
RNA was isolated using the NucleoSpin RNA kit (Mache-rey-Nagel) according to the manufacturer’s instructions. RNA was transcribed into cDNA using an oligo-dT-primer and Revert Aid Reverse Transcriptase (Thermo Fisher Scien-tific, Waltham, USA). Quantitative real time polymerase chain reaction (qRT-PCR) was performed using the des FAST SYBR Green Master Mix (Thermo Fisher Scientific, Waltham, USA) and the following oligonucleotides: hGAPDH (forward: 5΄ AGCCACATCGCTCAGACAC 3΄; reverse: 5΄ GCCCAATACGACCAAATCC 3΄), hIL6ST (forward: 5΄ GGGCAATATGACTCTTTGAAGG 3΄; reverse: 5΄ TTCCTGTTGATGTTCAGAATGG 3΄), hIL6 (forward: 5΄ CACCAGGCAAGTCTCCTCAT 3΄; reverse: 5΄ TCCAAAGATGTAGCCGCCCC 3΄), hIL6RA (forward: 5΄ GCTGAACT-- TGCTCCCGACAC 3΄; reverse: 5΄ AAGAGCCCCCTCAGCAATGT 3΄), hIL11 (forward: 5΄ GGACAGGGAAGGGTTAAAGG 3΄; re-- verse: 5΄ CTCAGCACGACCAGGACC 3΄), hIL11RA (forward: 5΄ GGCTATGGACACTCTGCTGC 3΄; reverse: 5΄ GCAGACACCAGGGCTGTAG 3΄), mGAPDH (forward: 5΄ AAGGTCATCCCAGAGCTGAA 3΄; reverse: 5΄ CTGCTTCACCACCTTCTTGA 3΄), mIL6ST (forward: 5΄ ACCTGCTCTGGGTGGAATGG 3΄; reverse: 5΄CGGCTTGTTTGAGGTACGCC 3΄), mIL6 (forward: 5΄ TCCAGTT- GCCTTCTTGGGAC 3΄; reverse: 5΄ GTGTAATTAAGCCTCCGACTTG 3΄), mIL6RA (forward 5΄ CATCTGTGAGTGGCGTCCGA 3΄; reverse: 5΄ TCGTTGTGGCTGGACTTGCT 3΄), mIL11 (forward: 5΄ CTGCACAGATGAGAGACAAATTCC 3΄; reverse: 5΄ GAAGCTGCAAAGATCCCAATG 3΄) and mIL11RA (forward: 5΄AATACCGACCAGCACAGCAT 3΄; reverse: 5΄ TGACTCGTACCGCGTGTG 3΄).
Data were analyzed using the QuantStudio 6 Flex RT-PCR System (Thermo Fisher Scientific, Waltham, USA). The 2-ΔCT method was used, and gene expression was determined relative to the GAPDH amount of the cells.
Statistical analysis
Data were analyzed by one-way ANOVA following Dunnett’s multiple comparison test. Data analyses were performed using GraphPad Prism version 10 (GraphPad Software, La Jolla California USA, www.graphpad.com). We performed a comparison of untreated cells with all treated conditions. Statistical significance was determined at a level of p < 0.05 and the p-values are shown in the respective diagrams of the figures. In addition, the statistical test used is described in the respective figure legends.
Results
Gastric cancer cell lines express only small amounts of IL-11R and IL-11
IL-6 binds to the IL-6R and IL-11 binds to the IL-11R. Both cytokines engage a homodimer of the signal-transducing β-receptor gp130 to activate intracellular signaling cascades in their respective target cells [10]. In order to analyze which cytokines might play a role in gastric tumors, we first analyzed expression of genes encoding the five proteins of interest in three different gastric cancer cell lines using qRT-PCR. In AGS cells, which is a cell line from a female patient with gastric adenocarcinoma, we could detect mRNA for IL6 (expression relative to GAPDH 0.05 ±0.02) and IL6R (relative expression 0.1 ±0.02) (Fig. 1 A). In contrast, the expression level of IL11RA was lower (relative expression 0.02 ±0.01), and IL11 itself was barely detectable. Due to the ubiquitous expression of gp130, it was expected that IL6ST could be robustly detected (relative expression 1.34 ±0.41) (Fig. 1 A). In order not to rely on a single cell line, we performed the same analysis with NCI-N87 cells, a cell line that was isolated from the stomach of a male patient with gastric carcinoma. We could again clearly detect IL6ST (relative expression 16.62 ±4.27) and IL6R expression (relative expression 0.9 ±0.08) (Fig. 1 B). However, expression of IL6, IL11 and IL11RA was barely detectable (Fig. 1 B).
Fig. 1
High levels of IL6ST in gastric tumor cell lines. A, B) Expression of IL6, IL11, IL6R, IL11RA and IL6ST was analyzed via quantitative real time polymerase chain reaction (qRT-PCR) in AGS cells (A) and N87 cells (B). Data are shown as mean ± SD relative to GAPDH expression and are derived from three independent experiments. C) Expression of Il6, Il11, Il6r, Il11ra and Il6st was analyzed via qRT-PCR in CLS-103 cells Data are shown as mean ± SD relative to GAPDH expression and are derived from three or four independent experiments.
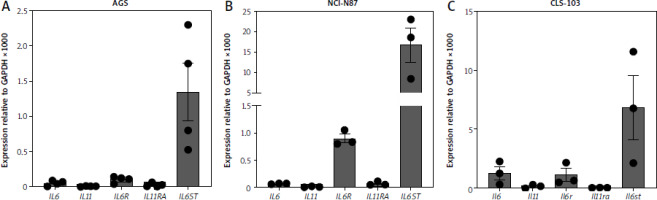
Because several of the studies regarding a functional role of IL-11 in gastric tumors have been performed in mice, we additionally did the same analysis with CLS-103 cells, a murine gastric squamous cell carcinoma cell line. As shown in Figure 1 C, CLS-103 cells expressed significant amounts of Il6 (relative expression 1.25 ±0.56), Il6ra (relative expression 1.09 ±0.54) and Il6st (relative expression 6.8 ±2.7), but only a small amount of Il11, while Il11ra was not detectable. We concluded from these experiments that high IL-11R expression is not a feature of these gastric cancer cell lines, and that this is species-independent. In contrast, the consistent expression of the IL-6R suggests that IL-6 rather than IL-11 signaling might be crucially involved in gastric tumorigenesis.
Gastric cancer cell lines respond poorly to stimulation with exogenous IL-11
Having shown that gastric cancer cell lines express little IL11R, but much more IL6R mRNA, we next sought to validate whether they could be activated by stimulation with exogenous IL-6 or IL-11. We performed these experiments because mRNA levels do not always accurately reflect the actual protein level, and we have shown previously that rather low levels of IL-11R are sufficient to activate intracellular signaling cascades when cells are stimulated with IL-11 [46, 47]. For this, we seeded AGS cells, serum-starved them for 24 hours and either stimulated them for 15 min with 10 ng/ml IL-6, 10 ng/ml IL-11, or 10 ng/ml Hyper-IL-6, or left them untreated. Hyper-IL-6 is a fusion protein of IL-6 and the soluble IL-6R which can directly activate a gp130 homodimer without the need of a membrane-bound IL-6R and serves as the positive control [43]. Afterwards, the cells were lysed and the phosphorylation of the transcription factor STAT3 was analyzed by western blot. As shown in Figure 2 A, AGS cells responded weakly to stimulation with IL-6 and IL-11 (2.4 ±1-fold and 1.6 ±0.3-fold increase compared to unstimulated cells, respectively), while phosphorylation of STAT3 was significant when the cells were stimulated with Hyper-IL-6 (6.5 ±3.8-fold increase, p = 0.0261) (Fig. 2 A). NCI-N87 cells responded well to IL-6 stimulation (20.8 ±9.7-fold increase compared to the unstimulated control), which was not the case for IL-11 stimulation (3.7 ±1-fold increase) (Fig. 2 B). We observed again the strongest STAT3 activation when cells were stimulated with Hyper-IL-6 (120.7 ±113.4-fold increase) (Fig. 2 B). We obtained similar results with the CLS-103 cell line, which also responded to IL-6 (3.4 ±1.1-fold increase compared to the unstimulated control) and Hyper-IL-6 (29 ±8.8-fold increase, p = 0.0002) stimulation, but not when stimulated with IL-11 (1.1 ±0.1-fold increase) (Fig. 2 C). We concluded from these experiments that the analyzed gastric tumor cell lines express functional IL-6R at levels that make them responsive to IL-6 stimulation, but that the IL-11R levels are too low to provoke a meaningful biological response when stimulated with IL-11.
Fig. 2
Gastric cancer cell lines respond to IL-6 trans-signaling. Serum-starved AGS cells (A), N87 cells (B) and CLS-103 cells (C) were either stimulated with IL-6, IL-11, or Hyper-IL-6 for 15 min or left unstimulated. Afterwards, cells were lysed and phosphorylation of the transcription factor STAT3 was analyzed by western blot. STAT3 and GAPDH were analyzed to verify equal loading. One representative experiment is shown, and the corresponding densitometric analysis of the pSTAT3/STAT3 ratio (mean ± SD, n = 3) is shown next to the western blots. Statistical analysis was performed using one-way ANOVA following Dunnett’s multiple comparison test. Here, all stimulated conditions were compared to the unstimulated cells and in case of statistical significance, the p value is shown above the respective bracket. All other comparisons were not significantly different
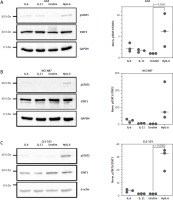
Immune cell lines express high levels of IL-6R
Immune cells play important yet not completely understood roles in gastric tumorigenesis [8, 48]. Because gastric tumor cell lines appear not to be the major targets of IL-11, we sought to elucidate whether immune cell lines might be targets of IL-11. For this, we first analyzed mRNA levels in THP-1 cells, a monocytic cell line isolated from a patient with acute monocytic leukemia, and U-937 cells, a pro-monocytic cell line isolated from a patient with histiocytic lymphoma. As shown in Figure 3 A and B, IL6R transcripts were highly abundant in these cell lines (relative expression 29.26 ±13.08 and 127.8 ±34.98 respectively), whereas IL6ST was detectable, but at lower levels (relative expression 0.64 ±0.09 and 2.74 ±0.73 respectively). IL6, IL11 and IL11R could not be detected. In order to again not only rely on human cell lines, we performed the same analysis with J774A.1 cells, a murine monocyte-macrophage cell line. These cells expressed high levels of IL6 (relative expression 0.7 ±0.5), Il6ra (relative expression 1.72 ±0.4) and Il6st (relative expression 2.33 ±0.63), but no Il11 and Il11ra (Fig. 3 C). We concluded that at least these three immune cell-derived cell lines are also not primary target cells of IL-11, but nevertheless wanted to verify this at the functional level. As done with the gastric tumor cell lines before (Fig. 2), we serum-starved the cells for 5–6 hours, stimulated them with the indicated cytokines for 15 min, lysed the cells and determined STAT3 phosphorylation as the readout. THP-1 cells responded to stimulation with IL-6 (143.9 ±65.5-fold increase, p = 0.0022) and Hyper-IL-6 (49.5 ±16.8-fold increase) by phosphorylation of STAT3, but did not respond to IL-11 stimulation (Fig. 3 D). Likewise, U-937 cells showed strong phosphorylation of STAT3 in response to IL-6 (77.2 ±17-fold increase, p = 0.0003) and Hyper-IL-6 (29.3 ±20.1-fold increase), but not in response to IL-11 stimulation (Fig. 3 E). This was again not restricted to human cell lines, as J774A.1 cells also responded to IL-6 (19.2 ±12.9-fold increase) and Hyper-IL-6 treatment (53.1 ±1 3.5-fold increase, p = 0.0004), but not to IL-11 stimulation (Fig. 3 F). We concluded that also in immune cell lines IL-6, and not IL-11, is the dominant cytokine that is able to activate the cells.
Fig. 3
Monocyte-derived immune cell lines express high levels of IL6R and respond to IL-6 classic and trans-signaling. A-B) Expression of IL6, IL11, IL6R, IL11RA and IL6ST was analyzed via quantitative real time polymerase chain reaction (qRT-PCR) in THP-1 cells (A) and U-937 cells (B). Expression of Il6, Il11, Il6r, Il11ra and Il6st was analyzed via qRT-PCR in J774.A1 cells. Data are shown as mean ± SD relative to GAPDH expression and are derived from three independent experiments (C). Serum-starved (D) THP-1 cells, (E) U-937 cells and (F) J774.A1 cells were either stimulated with IL-6, IL-11, or Hyper-IL-6 for 15 min or left unstimulated. Afterwards, cells were lysed and phosphorylation of the transcription factor STAT3 was analyzed by western blot. STAT3 and GAPDH were analyzed to verify equal loading. One representative experiment is shown, and the corresponding densitometric analysis of the pSTAT3/STAT3 ratio (mean ± SD, n = 3) is shown next to the western blots. Statistical analysis was performed using one-way ANOVA following Dunnett’s multiple comparison test. Here, all stimulated conditions were compared to the unstimulated cells and in case of statistical significance, the p-value is shown above the respective bracket. All other comparisons were not significantly different
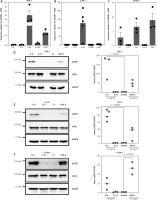
Expression of IL6, but not IL11 or IL11R, is predictive of overall survival in stomach adenocarcinoma patients
Our in vitro data so far suggest that IL-6 rather than IL-11 could potentially play a role in gastric tumorigenesis. In order to obtain further insight, we analyzed expression data from gastric cancer patients and controls using gene expression profiling interactive analysis 2 (GEPIA2) [42]. We analyzed the stomach adenocarcinoma (STAD) dataset, consisting of 408 tumor samples and 211 tissue-matched control samples. Interestingly, the tumor samples had significantly reduced amounts of IL11RA compared to the control tissue (Fig. 4 A). Amounts of IL11 were increased in the tumor tissue compared to the controls, but this did not reach statistical significance (Fig. 4 B). Afterwards, we used survival data and divided the patients into a low and a high expression group individually for both genes, resulting in 192 patients in each group (Fig. 4 C, D). In line with our previous findings, neither IL11RA (p = 0.31) (Fig. 4 C) nor IL11 expression (p = 0.66) (Fig. 4 D) had a significant influence on the overall survival of the patients. Afterwards, we analyzed expression of IL6R and IL6 in the same patient cohorts, which did not reveal any significant difference (Fig. 4 E, F). When we assessed overall survival by stratifying the patients again into a high and a low expressing group for both genes, we observed no difference when grouped according to IL6R expression (Fig. 4 G). However, the low IL6 group had a significantly better overall survival compared to the high IL6 group (p = 0.01) (Fig. 4 H). In summary, our expression analysis revealed no role for IL-11/IL-11R in overall survival of stomach adenocarcinoma patients, but showed that low levels of IL-6 were associated with better overall survival of these patients.
Fig. 4
Expression of IL6 in gastric tumors is associated with patient overall survival. A, B) Expression levels of IL11RA (A) and IL11 (B) in gastric tumor samples (red) and non-tumor control samples (gray). C, D) Tumor samples were divided into two groups (high/low) depending on their expression of IL11RA (C) or IL11 (D) and overall survival was analyzed for both groups. Expression levels of IL6R (E) and IL6 (F) in gastric tumor samples (red) and non-tumor control samples (gray). Tumor samples were divided into two groups (high/low) depending on their expression of IL6R (G) or IL6 (H) and overall survival was analyzed for both groups
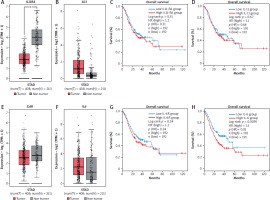
Discussion
Gastric cancer represents a major challenge to treat and therefore results in around one million deaths every year [1]. Although the number of affected patients has been decreasing in recent years, this is primarily due to a reduction in Helicobacter pylori infections, one of the major causes of gastric tumorigenesis. Curative treatment, especially of patients with further progressed disease, is still difficult with the currently available options. Thus, the identification of novel therapeutic targets that can be used to either prevent or treat gastric tumors is of high relevance.
In the present study, we found that three gastric cancer cell lines express high amounts of gp130, the shared signal-transducing receptor of the IL-6 family of cytokines, rather low levels of the IL-6R and basically no IL-11R. Accordingly, these cell lines respond to stimulation with recombinant IL-6, but much better when stimulated with Hyper- IL-6, a fusion protein that mimics IL-6 trans-signaling. As it has been demonstrated before that STAT3, the transcription factor downstream of gp130, is crucial for gastric tumorigenesis [49, 50], these results suggest that IL-6, and especially IL-6 trans-signaling, are required for STAT3 activation in the investigated gastric cancer cell lines.
Rather surprisingly, we found no strong expression of IL-11R mRNA in the three tumor cell lines, and accordingly these cells did not respond to stimulation with IL-11, as seen by no phosphorylation of the transcription factor STAT3. This is at odds with previous studies that demonstrated a crucial, non-redundant role for IL-11 in gastric tumorigenesis [40, 41, 51]. This might be explained by the fact that most of the previous studies have been performed in mice and not in human samples. However, we also observed no strong effect of IL-11 on the murine cell line CLS-103. Otherwise, these differences could also be attributed to the fact that the cell lines that we used do not accurately depict the whole spectrum of gastric tumor cells that one would see, e.g. in a tumor specimen directly derived from cancer patients, and suggest that IL-11 signaling could be more important in other cell types within the tumor or its environment, but not in the cancer cells themselves. Furthermore, Helicobacter pylori infection is not reflected in our cell culture model, an important factor that appears to contribute to IL-11-dependent gastric tumorigenesis [52].
Gastric tumors do not consist entirely of tumor cells, but also contain immune cells [8, 48]. Whether cytokines are derived from these invading immune cells, whether cytokines act on the immune cells or both is not entirely clear. We therefore analyzed whether different monocyte-derived immune cell lines respond to IL-6 and IL-11. Intriguingly, we observed high amounts of IL-6R and thus an efficient response to stimulation with exogenous IL-6, which is in line with previous reports that monocytes are among the cell types in the human body that can be activated by IL-6 via classic signaling [53–55]. Furthermore, these cells can be the source of sIL-6R to activate trans-signaling, as cells expressing IL-6R are likely candidates to release sIL-6R via proteolysis [22, 27]. Indeed, deletion of IL-6R via LysM-Cre resulted in 60% reduction of sIL-6R in mice, underscoring that these cells are important contributors to steady-state sIL-6R levels [56]. Again, we found no evidence for a prominent role of IL-11/IL-11R signaling in these cells.
Lastly, we analyzed differential gene expression data in gastric tumor patients compared to non-tumor controls and determined whether high or low expression levels would be predictive of overall survival. While we found no difference for IL11 or IL11RA, low levels of IL6 were associated with better survival. This corresponds to our in vitro data showing that gastric tumor cells respond better to IL-6 signaling compared to IL-11 signaling.
Conclusions
In summary, our data suggest that IL-6 contributes to gastric tumorigenesis. Our results highlight that the tumor cell lines we used do not accurately reflect the in vivo situation, as they are not responsive to IL-11. Future experiments should use primary tumor cells or organoids derived from primary tumors to better determine the individual contribution of IL-6 and IL-11 via classic and trans-signaling pathways in cancer and immune cells in gastric tumors.