Purpose
The standard of care in the curative treatment of locally advanced cervical cancer (LACC; stages, 1B2-4A) is external beam radiotherapy (EBRT), and intra-cavitary (IC) and/or interstitial (IS) brachytherapy (BT) boost with concomitant chemotherapy. Both radiotherapy modalities have been developing rapidly, with increasingly sophisticated techniques appearing that escalate the dose to the tumor and spare organs at risk (OARs) [1-7].
To reach this planning aims, BT evolved in terms of image techniques. Using computed tomography (CT), magnetic resonance imaging (MRI), ultrasonography (US), or fusion of these images, helps to determine the residual disease after EBRT, and irradiation of healthy tissues in the uterus is therefore not required [8-13].
On the other hand, the design and versatility of the applicators are also adapted to a new concept, where spatial anatomy of target volumes and OARs is well-known. Traditional tandem/ring or tandem/ovoid combined with IS-IC applicators are available, including Vienna, Utrecht, Venezia, Geneva, and 3D-printed [14-19]. While IC applicators historically were used with a pre-defined source loading and dose prescription method to specify the dose to points A (Manchester system), in the possession of 3D anatomical information, tailoring the dose to the target volumes became possible with individualized dose optimization [20, 21]. Otherwise, this transition cannot be smooth as dose to points A is not suitable for dose prescription when IS needles go through or directly next to the needles, increasing the dose to false value [22].
Advanced inverse optimization methods complicate the situation even more [23-25]. The question is: do we need to implement the historical point of view into the inverse algorithms, or change to a totally new concept? We can restrict the relative loading of intra-uterine, vaginal (ring/ovoid, R/O) part of the applicator and IS needles to reach the well-known pear-shape spatial dose distribution. Or else, we can use these advanced tools (imaging, applicator and optimization) to create a tumor-shape dose. The main difference in loading is the vaginal channel (R/O) of the applicator. In residual tumors without vaginal involvement, the applicator touches the surface of the cervix, so the source can irradiate the target volume from outside only. Mohamed et al. tried to reduce the vaginal dose by decreasing dwell times in R/O and increasing dwell times in tandem/needles [26]. However, not loading of channel of the applicator is still an unsolved question.
The aim of the present study was to examine the effect of inactivate R/O channel of IC and combined IS-IC applicators in term of dose coverage and conformity of the target volumes and doses to all OARs, when high-risk clinical target volume (HR-CTV) does not extend to the R/O applicator. Fulfilment of the criteria of international recommendations for targets and OARs was investigated.
Material and methods
At our institute, sixty patients with cervical cancer without vaginal involvement (stage, IB2-IVA) were selected, who received 7 Gy high-dose-rate BT in each of 4 implantations, following 50.4 Gy EBRT with intensity-modulated arc therapy for HR-CTV. Patients were examined with pelvic MRI and staged with CT or PET-CT at the beginning, and the therapeutic effect was assessed with an MRI at the end of EBRT. Initial and post-EBRT MRI were used to determine the number and position of the needles in the ring or Venezia applicator, if needed [18-20]. Interstitial BT was considered for patients, whose disease could not be adequately encompassed by IC application. Thirty patients had combined IC-IS application, and in other 30 cases, only IC insertion was applied. Delineation of gross tumor volume (GTV), HR-CTV, intermediate-risk clinical target volume (IR-CTV), bladder, rectum, sigmoid, bowel, and vaginal wall was performed following the GEC-ESTRO recommendations for CT-based contouring [21]. Post-EBRT MRI and post-implant CT series were placed side-by-side in the same monitor, and with the help of an expert radiologist, the contours were created on post-implant CT using information of post-EBRT MRI. To avoid inter-observer variability and reduce contouring bias, the same expert radiation oncologist created the contours in all the cases. The distance between the cervix and the surface of R/O was 10.8 mm in average (range, 4.2-24.5 mm), according to the given cervical anatomy (scab, deformity, etc.) and vaginal stenosis after EBRT. As the vagina was not involved in these patients, none of the target volumes contained the vagina and this air gap. Therefore, necessity of active source dwell positions in R/O part of the applicators – outside of HR-CTV was questionable. Two different treatment plans were created for each 60 patients, with and without activation of the R/O part of IC or IS applicator, using the same planning aims. In all of these cases, the plan with inactive R/O was applied for the treatment of patient. During treatment planning, manual optimization was used to achieve the optimal dose distribution (Oncentra Brachy version v. 4.5.3, Elekta Brachytherapy, Veenendaal, The Netherlands). At first, geometrical optimization was applied in distance mode. Then, where the reference doses did not cover target volumes (GTV and HR-CTV), the reference isodose lines were expanded graphically or an appropriate dwell times were improved manually based on their distance from OARs. In the opposite cases, where the target volumes were covered with an appropriate dose but OARs were over-exposed, isodose lines or dwell times of the source were also modified. The planning aim was to fulfil the criteria of the recommendations of GEC-ESTRO Gynecological Working Group [28, 29], the EMBRACE II study [30] and ABS [31, 32].
EQD2 total doses of EBRT plus BT plans were calculated using linear-quadratic radiobiological model [33, 34]. α/β of HR-CTV was 10 Gy, while for OARs, 3 Gy was applied. The following dose-volume parameters were used for quantitative evaluation of plans [35]:
V150: relative volume of IR-CTV, HR-CTV, and GTV receiving at least 150% of the prescribed dose (%),
D90, D98: minimum dose delivered to 90% and 98% of IR-CTV, HR-CTV, and GTV (Gy),
DA: dose to point A (Gy),
DNR: dose non-uniformity ratio [36],
COIN: conformal index [37],
D2: minimal dose of the most exposed 2 cm3 of a critical organ, including the bladder, rectum, sigmoid, bowel, and vaginal wall (Gy),
DICRU: dose to ICRU reference point of the bladder and rectum (recto-vaginal point) (Gy) [38],
DPIBS: dose to the point of posterior-inferior border of the symphysis (Gy),
DPIBS+2: dose to the point 2 cm above posterior-inferior border of the symphysis parallel to applicator axis (Gy),
DPIBS-2: dose to the point 2 cm below posterior-inferior border of the symphysis parallel to applicator axis (Gy).
EQD2 dose-volume parameters between the competing plans were compared using Wilcoxon-matched pairs test (Statistica v. 12.5, StatSoft, Tulsa, OK, USA).
Although, this was a dosimetric study, clinical data of the limited patients’ cohort were also assessed.
Results
The mean volume of HR-CTV after EBRT (residual tumor volume) was 35.3 cm3 (range, 8.7-100.2 cm3), while IR-CTV was 102.4 cm3 (range, 44.2-227.9 cm3) and GTV was 15.7 cm3 (range, 0.0-54.0 cm3). These volumes were 39.6 cm3 (range, 19.4-100.2 cm3), 136.1 cm3 (range, 44.2-227.9 cm3), and 16.8 cm3 (range, 0.0-54.0 cm3) in IS, and 18.8 cm3 (range, 8.7-31.8 cm3), 88.9 cm3 (range, 79.8-95.9 cm3), and 11.3 cm3 (range, 0.0-33.7 cm3) in IC applications. In IS implantations, median 4 needles (range, 3-12) were used additionally to the IC applicator.
There was no significant difference in the dose of HR-CTV between the plans with inactive vs. active R/O (mean D90: 91.2 vs. 91.5 Gy; mean D98: 80.6 vs. 81.0 Gy). Similar results were obtained for GTV (D90: 115.3 vs. 109.1 Gy; D98: 97.2 vs. 95.5 Gy). The mean D98 of IR-CTV was significantly lower with inactive R/O (60.8 vs. 63.9 Gy, p = 0.0464); however, the GEC-ESTRO (EMBRACE II study) and ABS criteria were fulfilled in 96% in each of the plans. There was no difference in dose homogeneity (DNR: 0.55 vs. 0.54), but the conformity of the plans with inactive R/O was considerably higher (COIN: 0.62 vs. 0.38, p < 0.001).
Doses to all OARs were significantly lower in plans without R/O activation: D2 (bladder): 72.4 vs. 86.5 Gy (p < 0.001); D2 (rectum): 57.9 vs. 75.2 Gy (p < 0.001); DICRU(recto-vaginal): 58.8 vs. 77.5 Gy (p < 0.001); D2 (sigmoid): 61.7 vs. 67.3 Gy (p < 0.001); D2 (bowel): 69.2 vs. 72.1 Gy (p = 0.0038); and D2 (vaginal wall): 78.2 vs. 117.3 Gy (p = 0.0022) in plans with inactive vs. active R/O, respectively. The same trend was observed in the dose of points on the vaginal wall: DPIBS was 50.6 vs. 53.0 Gy (p < 0.001), DPIBS+2 was 53.1 vs. 57.7 Gy (p < 0.001), and DPIBS-2 was 49.5 vs. 50.7 Gy (p < 0.001), respectively (Table 1).
Table 1
EQD2 total doses of dose plans with inactive vs. active ring/ovoid (R/O) channel of the applicator in high-dose-rate brachytherapy of cervical cancer
[i] V150 – the relative volume of intermediate-risk CTV (IR-CTV), high-risk CTV (HR-CTV), and GTV receiving at least 150% of the prescribed dose (%); D90, D98 – the minimum dose delivered to 90% and 98% of IR-CTV, HR-CTV, and GTV (Gy); DA – dose to point A (Gy); DNR – dose non-uniformity ratio; COIN – conformal index; D2 – minimal dose of the most exposed 2 cm3 of organs at risk (bladder, rectum, sigmoid, bowel, and vaginal wall) (Gy); DICRU – dose to ICRU reference point of bladder and rectum (recto-vaginal point) (Gy); DPIBS – dose to the point of posterior-inferior border of the symphysis (Gy); DPIBS+2 – dose to the point 2 cm above from posterior-inferior border of the symphysis (Gy); DPIBS-2 – dose to the point 2 cm below from posterior-inferior border of the symphysis (Gy); * Wilcoxon-matched pairs test
Evaluating separately the IS and IC only applications, the same trends appeared between plans using inactive versus active R/O (Tables 2 and 3). Only the difference in D90 of GTV between inactive versus active R/O plans was almost statistically significant in IS applications: 113.5 Gy vs. 106.5 Gy (p = 0.0531). However, this difference was 7 Gy EQD2 only, which was clinically insignificant. It has to be noted, that activating the R/O part of the applicator increased D2 and DICRU of the bladder and D2 of the rectum in higher degree in IC applications (+19.0 Gy, +21.6 Gy, and +19.4 Gy, respectively) compared with those in IS (+12.7 Gy, +10.1 Gy, and +14.7 Gy, respectively) (p < 0.001). There was no significant difference in the changing of other examined dose-volume parameters by R/O activation between the IS and IC only applications.
Table 2
EQD2 total doses of dose plans with inactive vs. active ring/ovoid (R/O) channel of interstitial-intra- cavitary applicator in high-dose-rate brachytherapy of cervical cancer
Table 3
EQD2 total doses of dose plans with inactive vs. active ring/ovoid (R/O) channel of intra-cavitary applicator in high-dose-rate brachytherapy of cervical cancer
It was found that HR-CTV was exposed at least with the recommended EQD2 total dose (D90) in 100% of the patients with inactive and active R/O techniques. The criteria for the D98 parameter were fulfilled in 96% of the plans without activate R/O, and in 92% using active R/O. GTV was covered with D98 dose in 100% of the cases in plans with inactive R/O, and in 80% with active R/O. However, the D98 dose coverage of IR-CTV was the same in each of the plans – 96%. Only the EQD2 dose criteria of the points A was fulfilled less without active R/O, showing 88%. While using active dwells in R/O, 100% of the plans fulfilled this criterion.
Even though all the plans without R/O activation fulfilled the GEC-ESTRO (EMBRACE II study) and ABS dose criteria (D2) in 100% for the bladder, rectum and sigmoid, the plans with activated R/O complied with the same criteria in 72%, 56%, and 88%, respectively (Table 4).
Table 4
Percentage of dose plans using inactive vs. active ring/ovoid (R/O) channel of the applicator, which fulfilled the criteria of the GEC-ESTRO (EMBRACE II study) and ABS recommendations (hard/soft constraints)
These proportions were similar in the IS and IC applications when analyzed separately; however, the effect of R/O activation was slightly higher in IC only cases. The ratio of the plans that fulfilled the criteria were lower in almost all of the examined parameters in IC applications than IS cases using active R/O (Tables 5 and 6).
Table 5
Percentage of dose plans using inactive vs. active ring/ovoid (R/O) channel of interstitial-intra-cavitary applicator, which fulfilled the criteria of the GEC-ESTRO (EMBRACE II study) and ABS recommendations (hard/soft constraints)
Table 6
Percentage of dose plans using inactive vs. active ring/ovoid (R/O) channel of intra-cavitary applicator, which fulfilled the criteria of the GEC-ESTRO (EMBRACE II study) and ABS recommendations (hard/soft constraints)
In the cohort of the present study, the median follow-up time was 64 months. The local control rate was 97.6% (2 patients had local recurrence), 4 patients had distant metastases, and 2 patients died during this time. No necrosis was reported.
Discussion
Brachytherapy boost has a fundamental role in the radiotherapy of LACC. It is used to guarantee local control with irradiation of residual tumor with appropriate dose, apart from avoiding treatment-related morbidity with sparing dose to OARs [6]. Evidence confirm its effectiveness in local control; the EMBRACE study has reported rates of over 90% for all FIGO stages at 3 years [30, 39, 40]. However, various severe toxicities were also reported, with most serious including rectal fistula and vaginal stenosis. As certain dose-volume parameters of the target volumes correlate to local control [41, 42], the dose parameters of these organs predict the prevalence of side effects [43, 44]. Recently, the main question is: how we can reach the optimal value of these dose-volume parameters?
In the era of advanced imaging modalities, special IS-IC applicators with the opportunity of oblique needles and inverse dose optimization methods, there is no strong evidence available for implementing one approach over another. Tanderup et al. recommended dose planning aims and dose prescription in image-guided cervical BT [45]. However, these conclusions were based on the clinical evidence of treatment plans using the traditional pear-shape spatial dose only. Earlier, the same team tried to de-escalate the vaginal dose by decreasing dwell times in R/O and increasing it in tandem/needles [27]. The aim was to maintain the target dose while reducing the dose to the surface of the vagina to be < 140% of the physical fractional BT dose corresponding to a total EQD2 of 85 Gy. The R/O loading was reduced from 51% to 33% of total loading, which resulted in a reduced dose to ICRU recto-vaginal point by a mean of 4 ±4 Gy EQD2, and D2 doses to the bladder and rectum were reduced by 2 ±2 Gy and 3 ±2 Gy, respectively. In the current study, we achieved the total dose de-escalation of the critical tissues, as source loading in the R/O channel of the applicators was reduced to zero in cases where residual tumors did not involve the vagina. This approach simply follows the basic rule of BT that active dwell positions have to be inside or on the surface of the target volume. This technique results in a highly conformal tumor-shape dose distribution around the target volumes, breaking the rules of the conventional pear-shape dose (Figure 1).
Fig. 1
Isodose surface (light red) of the prescribed dose (top) and dose distribution (bottom) without (left) and with using active dwells in the ring/ovoid of the applicator (right). Pink – IR-CTV; Red – HR-CTV; Grey – GTV; Yellow – bladder; Dark green – rectum; Light green – sigmoid
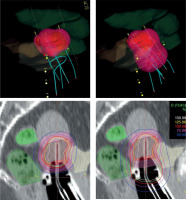
While dose coverage of the target volumes is similar to using active R/O, the EQD2 total D2 dose to the bladder, rectum, sigmoid, small bowel, and vaginal wall were reduced by 14.1 Gy, 17.3 Gy, 5.6 Gy, 2.9 Gy, and 39.1 Gy, respectively. However, the dose to the IR-CTV was smaller without loading the R/O (D98 were 60.8 Gy using inactive, and 63.9 Gy with active R/O); these dose plans fulfilled the GEC-ESTRO (EMBRACE II study) and ABS recommendations in the same degree, i.e., 96%. In terms of residual GTV, the D90 non-significantly increased with inactive R/O (97.2 Gy vs. 95.5 Gy). Loading R/O shifts higher doses to the direction of R/O, where there is no GTV, and accordingly, unnecessarily increases the dose to OARs. The loading patterns also illustrate this effect. The tandem dominates the contribution to TRAK between the tandem and needles without R/O loading (53.2% vs. 40.8%), and these shifts to a harmonized distribution with R/O activation (Figure 2). Dose to points A is not interpretable, because of the proximity of IS needles. With the same level of homogeneity, inactive R/O results in almost 2 times higher dose conformality of the target volumes (COIN: 0.62 vs. 0.38).
Fig. 2
Contribution of TRAK between tandem, ring/ ovoid (R/O) and needles of cervical brachytherapy applicators using inactive and active R/O channel in interstitial- intra-cavitary applicator
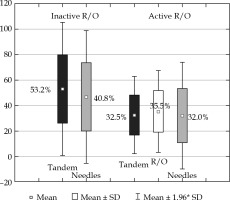
The planning aim in both dose plans with inactive or active R/O was to fulfil the GEC-ESTRO (EMBRACE II study) and ABS dose recommendations. The HR-CTV was exposed at least with the recommended EQD2 total dose (D90) in 100% of the patients with inactive and active R/O techniques. Criteria for the D98 parameter were fulfilled in 96% of the plans without active R/O, and in 92% using active R/O. The GTV was covered with D98 dose in 100% of the cases in plans with inactive, and in 80% with active R/O. However, the D98 dose coverage of IR-CTV was the same in both the plans, i.e., 96%. Only the dose criterion of points A was fulfilled to a lesser extent without active R/O, i.e., 88%. While using active dwells in R/O, 100% of the plans fulfilled this criterion. While all the plans without R/O activation fulfilled the dose criteria (D2) in 100% for the bladder, rectum, and sigmoid, the plans with active R/O satisfied the same criteria in 72%, 56%, and 88%, respectively. Reducing R/O loading to zero showed better optimal dose plan as recommended by the GEC-ESTRO (EMBRACE II study) and ABS.
In IS applications, dwell positions in the needles can replace the dwells of R/O if needed, and can shape the dose distribution to conformal. Using R/O without active dwells is a recognized routine in IS applications; several centers use special (i.e., 3D-printed) template instead of the original R/Os [14-18]. This question is more interesting in the case of IC only applications, where without R/O, only a one-channel applicator has a rule in shaping the appropriate dose distribution. Since IC applications is applied for irradiation of smaller and symmetrical HR-CTVs, the cylindrical dose distribution from this one channel can cover the tumor conformally. Moreover, the reason can be the same that activating the R/O part of the applicator increases the dose to the bladder and rectum in higher degree in IC only applications compared with IS only applications. That is why the ratio of the plans fulfilled dose-volume criteria and were lower in IC applications than IS cases using active R/O.
Basically, the distance of the R/O source path from HR-CTV and from OARs is a good starting point to decide the activation of R/O. If the surface of the R/O part of the applicator touches the inferior border of HR-CTV, the former distance is 5-10 mm, depending on the applicator used. Especially in the case of a narrow vagina, an air gap can exist between R/O and the surface of HR-CTV (Figure 1), which may affect the use of vaginal component. It strongly influences dosimetric parameters, mainly dose conformality of the plans. If after dose optimization the dose exposition is higher to an OAR than to HR-CTV, an alternative dose plan is recommended without activation of R/O as well as evaluation of the fulfilment of dose recommendations in both the plans. The necessity of loading R/O strongly depends on an individual anatomy of a patient. Of note, in case of tumors with vaginal involvement, when HR-CTV includes a portion of the vagina, source dwell positions in R/O (in HR-CTV) has to be activated accordingly the basic rules of BT. However, majority of residual tumors of the cervix does not involve the vagina. In this case, the R/O part of the applicator can be only a template for IS needles, or a fixation tool for the intra-uterine tandem.
Nevertheless, the reported clinical data of the current dosimetric study, which focused on the improvement of the dose-volume parameters that were in correlation with tumor control and side effects, are promising, despite rather limited cohort of 60 patients. Reducing dose to OARs, especially to the vaginal wall, without compromising the dose coverage of the target volumes carries itself the promise of reducing the development of rectal fistula and vaginal stenosis, and therefore contribute to preservation of sexual function. Therefore, this study can be a starting point for a prospective clinical study to explore the clinical relevance of this dose planning method.
Conclusions
Inactivation of the R/O applicator results in similar dose coverage of the target volumes with lower doses to all OARs as activating R/O in cervix cancer patients when HR-CTV does not extend to the R/O applicator. The use of active source positions in the R/O performs worse regarding the fulfilment of the criteria of the international recommendations for OARs.