Purpose
Patients diagnosed with gynecologic (GYN) cancers are often indicated for treatment with radiotherapy. A substantial subset of these patients is treated with both external beam radiation therapy and high-dose-rate brachytherapy (HDR-BT). For patients with primary disease of the cervix, endometrium, or vagina as well as complex recurrences, HDR-BT may be delivered by perineal template interstitial procedures. These procedures are complex and, due to the variation in target volume location, require individualized dosimetry that is often compromised, compared with well-established trial outcomes where majority of patients are treated for less advanced disease with standard applicators to deliver brachytherapy [1, 2]
In treatment with standard intra-cavitary brachytherapy applicators for cervical cancer, such as tandem and ring applicators, toxicity to normal tissue structures is comprehensively reported [1, 3]. In many perineal template-based implants, doses to uninvolved normal tissue structures, such as the bladder and rectum are similarly reported [2], as the planned dose gradients from catheters to normal tissues may be similar to standard brachytherapy applicators. For the vaginal mucosa, perineal template interstitial implant procedures may be indicated for a broader selection of gynecologic cancers, which are not dosimetrically similar. These implants often have needles directly abutting or penetrating the vaginal tissue. To deliver dose to target, brachytherapy sources dwell within the thin catheter lumen that contacts the mucosa, resulting in higher doses than is typical from standard brachytherapy applicators.
Vaginal mucosa dose in GYN HDR-BT is not standardized and not specified in the most recent consensus guidelines from recognized bodies, such as the American Brachytherapy Society (ABS) [2, 3] and GEC-ESTRO GYN working group [4, 5]. Vaginal toxicity is an ongoing area of investigation, and it has been correlated with surrogate dose points reported in the literature. The ICRU Report 89 [6] recommends the recto-vaginal reference point dose as well as the posterior-inferior border of symphysis (PIBS) and PIBS ±2 cm points. These reference points are included in the planning aims and dose reported in the EMBRACE II study. In intra-cavitary and hybrid intra-cavitary/interstitial applicator treatment, a dose-effect relationship has been demonstrated between recto-vaginal reference point and grade two vaginal stenosis [7]. Both the recto-vaginal reference point and PIBS points are defined with respect to a conventional applicator and with a single channel centered in the vagina, which have radial dose distributions, and therefore point dose estimation is representative of circumferential dose within the vagina. However, the recto-vaginal reference point and PIBS points cannot be directly translated in the case of template interstitial HDR-BT, where these points do not represent predictable and representative dosimetry.
Alternatively, several other groups have taken the approach of dose-volume histogram (DVH) statistics to calculate vaginal mucosa hot spots [8-14]. Many of these studies used hot spot doses of all or a combination of D0.1cc, D0.5cc, D1cc, and D2cc [9-14], and defined the vagina as the volume extending to a depth of 1 to 5 mm from the surface of the vaginal applicator [9, 11, 12], or the full vagina as seen on computed tomography (CT) or magnetic resonance imaging (MRI) [8, 10, 13, 14]. In these intra-cavitary applicator-based gynecological brachytherapy studies, these hot spot metrics have been correlated with vaginal toxicity [8, 13, 14]. For example, Susko et al. found that for ring and tandem and for tandem and ovoid HDR-BT patients, a threshold dose of 108 Gy to D2cc of the vaginal mucosa differentiated patients experiencing vaginal toxicities, where at two years, 36.2% and 70.5% of patients experienced grade 2 or greater vaginal toxicities below and above the 108 Gy threshold, respectively, and 9.9% and 30.0% experienced grade 3 or greater toxicities, respectively [14]. DVH-based vaginal dose-effect relationships have been found for intra-cavitary techniques, but have not been investigated for interstitial techniques explicitly, which may be important given that representative vaginal dose reporting was lacking for these patients.
Vaginal dose evaluation at points or small volumes is problematic for interstitial brachytherapy techniques due to the very steep dose gradient and consequently, the uncertainties introduced when evaluating these metrics. The aim of the present work was to identify small volume DVH metrics that reliably report the high-dose to the vaginal mucosa. A reliable dose metric is one that is consistent in different dose calculation methods.
Across all studies and standard clinical practice, the American Association of Physics in Medicine (AAPM) Task Group 43 [15] (TG43) dose calculation is used to report dose. It is generally accepted that the differences in dose accuracy are likely to have minimal clinical impact between model-based dose calculation algorithms or Monte Carlo (MC) simulation compared with TG43 for GYN treatments [16-19]. Previous investigations have focused on target coverage and the rectum, bladder, and sigmoid doses. Investigations have not clearly examined vaginal dose hot spots or dose comparison in these treatment areas with a highest dose gradient area. Most studies concentrated on applicator-based techniques [16, 18, 19]. One study reporting the results of MC simulation for GYN perineal template interstitial HDR-BT procedures, did not examine vaginal dose [17].
AAPM Task Group 186 [19] provides the framework for evaluating model-based dose calculation algorithms (MBDCAs) in brachytherapy and quantifying performance compared with standard TG43 formalism. In this report, a 2% agreement tolerance is recommended between the MBDCAs and consensus TG43 parameters under reference conditions, and discrepancies above 2% should be carefully investigated.
In the current work, we evaluated vaginal dose in GYN perineal template interstitial HDR-BT treatments using MC simulation. This is important to characterize that an appropriate dose reporting strategy may be developed as vaginal toxicity is correlated to small volume dose metrics, and interstitial HDR-BT treatments have high-dose gradients in the vagina.
Material and methods
Patient and treatment characteristics
Gynecologic perineal template interstitial HDR-BT patients treated from September 2016 to November 2022 at the study institute were included in the study. All implants were performed using Syed/Neblett GYN templates and flexi-needles (Best Medical International, Springfield, VA, USA) under ultrasound guidance. Each patient received post-implant MRI/CT imaging for treatment planning. CT image resolution was 0.3 × 0.3 × 1.5 mm3. Treatment planning was performed using Oncentra Brachy v. 4.6 (Elekta, Stockholm, Sweden). Structures routinely contoured included gross tumor volume (GTV), clinical target volume (CTV), intermediate-risk clinical target volume (IR-CTV), bladder, rectum, and sigmoid. For this study, vaginal contours were created retrospectively in the original, clinical structure sets by expanding vaginal cylinder by 5 mm and removing the cylinder (vagina5mm). This contour was a simple geometric expansion that may include target volume. An additional normal tissue vaginal contour was created by subtracting CTV from vaginal expansion contour (vagina5mm-CTV). Figure 1 shows the vagina5mm contour and CTV for a representative patient CT with interstitial implant. Oncentra DVH calculation settings were set to the highest granularity: 800 bins, 200,000 sample points, and high-dose limit of 2× dose prescription. The high-dose limit was incrementally increased to 4×, 8×, and 16× as needed, when small volume dose metrics exceeded dose limit.
Monte Carlo simulations
Computed tomography images with clinically approved structure sets, new vaginal contours, and clinical treatment plans were exported and used to simulate MC-based dose distributions in EGS-NRC brachytherapy package egs_brachy [20]. Contoured structures were assigned tissue compositions, and are shown in Supplementary information (Table S1). Tissue compositions used are specified in ICRU 46 [21], except for cylinder material, Delrin® (Piedmont Plastics, Charlotte, NC) or homopolymer polyoxymethylene, which has a density of 1.41 g/cc [22], and air was set as defined in the TG43 report [15]. The air and lumen of interstitial needles in the implant were not modeled. For the rest of the body, egs_brachy assigned voxels to either air, female soft tissue, or cortical bone based on voxel CT number.
For the MC simulation, volume corrections were applied to remove iridium-192 (192Ir) source volumes from patient volumes. Dose calculation grid size was the same as the CT image matrix size (0.3 × 0.3 × 1.5 mm3). For each patient plan, 1 × 109 histories were simulated. Across all prescriptions, physical doses were converted to equivalent dose in 2 Gy fractions (EQD2) using an α/β = 10 for targets and α/β = 3 for OARs. The total EQD2 dose was reported to include external beam radiation therapy dose (typically 45 Gy in 25 fractions). Egs_brachy simulations were performed once with tissues modeled and once with all media set to water (MC and MCwater, respectively) to distinguish between dosimetric differences observed when modeling heterogeneous media versus homogeneous water using the same dose calculation software.
Dose reporting and data analysis
Since vaginal dose DVH metrics are not well-defined for GYN perineal interstitial HDR-BT, hot spot dose metrics were calculated in Oncentra (referred to as TG43) and MC simulations as the minimum dose to 0.1 cm3, 0.5 cm3, 1 cm3, 2 cm3, and 4 cm3 (D0.1cc, D0.5cc, D1cc, D2cc, and D4cc respectively). Standard DVH metrics were calculated for other relevant structures as well: D50, D90, and D98 for high-risk CTV; D90 and D98 for intermediate-risk CTV; D90 and D98 for GTV; D0.1cc and D2cc for the bladder, rectum, and sigmoid.
Dose differences were reported between TG43 and MC, TG43 and MCwater, and MC and MCwater to isolate differences due to modeling heterogeneous tissue versus homogeneous water, and due to different calculation software when the media was homogeneous water. Across the patient cohort, all dose metrics were reported as average and standard deviation in both absolute difference and percent difference relative to each calculation method.
Results
Patient and treatment characteristics
Between September 2016 and November 2022, 61 GYN perineal template interstitial HDR-BT patients were treated at the study institution and included in this research. Of the 61 patients, the primary disease sites included 32 cervical, 11 vaginal, and 18 endometrial. Across all site groups, the most common prescriptions were 24 Gy in 3 fractions (n = 33) and 18 Gy in 3 fractions (n = 20). The remaining nine patients had prescribed doses and fractionation in the range of 5 to 7.75 Gy in 3 to 6 fractions, respectively. For the 61 patients, a total of 82 separate clinically treated plans existed to account for adapted plans or new implants for subsequent fractions. A total of 164 total simulations were completed, once for heterogeneous and once for homogeneous water [23]. The average ± standard deviation number of needles used in these implants was 18 ±5, which universally included seven needles in the cylinder (six peripheral and one central).
Vaginal dose reporting
Dose differences between TG43 and MCwater are shown in Figure 2 for a representative patient. Figure 2 includes structure volumes and dose distributions on CT image datasets (Figure 2A) as well as dose maps calculated in MCwater (Figure 2B) and TG43 (Figure 2C). Clear discrepancies in the dose distributions were observed as the MC software removed dose inside the cylinder and sources, which is explained in more detail in the Discussion section.
Fig. 2
Axial and sagittal CT slices of the implant with the largest difference between TG43 and MCwater vagina5mm D2cc. Isodose lines are relative to 8 Gy prescription dose, B) MCwater, and C) TG43 calculated dose on the same axial slice in (A). MC and MCwater simulations do not calculate dose inside the cylinder and sources
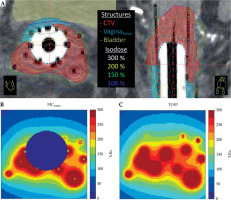
Dose differences between MC, MCwater, and TG43 for the vaginal expansion and representative normal vaginal tissue contour (vagina5mm and vagina5mm-CTV) are reported in Table 1 and Figure 3. For both MC and MCwater, the mean vaginal dose was less than TG43, indicating that standard clinical distributions over-estimated the vaginal hot spot dose. In general, the discrepancy between MC simulations and Oncentra was the greatest for the smaller volume DVH metrics, and the discrepancy decreased as the volume increased. For the vagina5mm-CTV D2cc and D4cc, and vagina5mm D4cc, the mean ± one standard deviation dose differences between MCwater and TG43 were within ± 5%, demonstrating reasonable agreement for dose volume metrics conventionally used to report normal tissues doses in GYN HDR-BT. Of the 61 patients simulated, an agreement between MCwater and TG43 was within ±5% for 62.3% and 86.9% of patients for the vaginal expansion (vagina5mm) D2cc and D4cc, respectively, and 83.6% and 98.4% of patients for the vaginal normal tissue (vagina5mm-CTV) D2cc and D4cc, respectively.
Fig. 3
Relative dose difference between MCwater and TG43 for each vaginal DVH metric. Dashed lines indicate ±5% range. The interquartile range for the vagina5mm-CTV D2cc and D4cc and the vagina5mm D4cc were within ±5% agreement. Whiskers represent the range, while outliers are data points outside 3× interquartile range
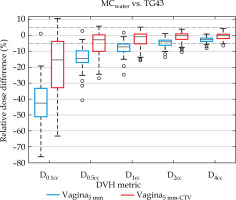
Table 1
Percentage of dose differences between MC, MCwater, and TG43 calculation methods, averaged over all patients in the cohort. Absolute doses calculated in TG43 are given for reference
Mean dose differences for all vaginal DVH metrics (Table 1) were 1.1% to 1.4% less in MC than MCwater, which was considerably less than the differences of over 4% observed between MC and TG43, revealing that the largest differences in clinically reported dosimetry was not due to the presence or absence of tissue modeling. In all other structures, MC and MCwater differences in mean DVH metrics (shown in Figure 4) were within 1%, consistent with previously published results [17].
Fig. 4
Relative dose difference of D2cc for OAR structures between MCwater relative to TG43. Boxes show interquartile range and whiskers indicate the range, while outliers are points outside 3× interquartile range
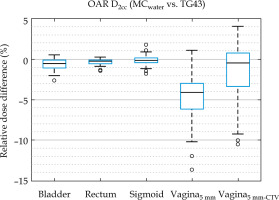
Correlation between the MCwater and TG43 dose for vagina5mm D0.1cc and D2cc is presented in Figure 5. TG43 and MCwater demonstrated a strong linear correlation (R2 = 0.989) for D2cc. For the smallest point volume (D0.1cc), the linear correlation was weaker (R2 = 0.561) showing that the relationship between TG43 and MCwater vagina5mm D0.1cc was less reliable.
Fig. 5
MCwater vs. TG43 dose for vagina5mm D0.1cc (A) and D2cc (B). Black dotted line is x = y. Linear fits are given for both distributions. Considerable scatter is observed with D0.1cc and worsens as dose increases, but when DVH volume is increased to D2cc, the relationship between MCwater and TG43 dose is much more correlated
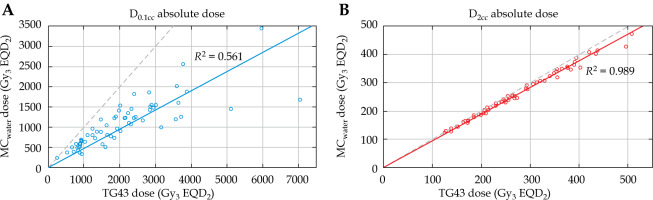
Monte Carlo statistical uncertainty
Average statistical uncertainty for all vaginal DVH metrics, averaged over all 176 simulations (1 × 109 histories), was less than 0.8%. The largest statistical uncertainty for the vagina5mm and vagina5mm-CTV metrics for a single patient were 1.0% and 2.2%, respectively, both for D4cc. Average statistical uncertainty for all other DVH metrics were less than 1.5%.
Discussion
This study investigates the dose reported to the vaginal mucosa for clinical GYN perineal template interstitial HDR-BT treatments. Due to interstitial nature of the technique, the reported doses are much different compared with standard GYN HDR-BT applicator treatments. Differences between MC-based dose and TG43-based dose were compared to evaluate discrepancies in conventional clinical dose reporting. For vaginal dose-volumes, the 2 cc represents the smallest volume with the expected correlation and within 5% average accuracy between dose calculation algorithms. The large uncertainties in small dose volumes result in unreliable plan quality evaluation, which may contribute to previous dose-effect relationship studies not determining correlations for volumes below D2cc [14].
Independent investigation is required because the previously established dose-effect relationships are not representative of the dose delivered to the mucosa for interstitial procedures that is established in the report of this study. For template interstitial implants, the doses can be particularly high when the target volume is in or nearby the conventional vaginal mucosa structure due to the proximity of needles that must be loaded to achieve adequate therapeutic dose to the target. The combination of small DVH metric volumes and high-dose gradient regions leads to challenging dosimetry. To establish dose-effect relationships for doses that may be delivered in interstitial procedures, a reliable dose metric is required for dose reporting. In this investigation, volumes of 0.1 cc, 0.5 cc, and 1 cc yielded poorly correlated estimates, and only evaluating 2 cc and 4 cc volumes produced accurate and reproducible dosimetry.
The authors acknowledge that vaginal dose may not be a high priority for planning in interstitial HDR-BT, especially when the tumor resides within the vaginal mucosa directly, and the primary goal is to cover the target with margins considering uncertainties at the expense of delivering dose to the healthy vaginal tissue. However, vaginal doses should still be reported to indicate potential severe toxicities. Given the complex dose distributions and lack of standard and representative dose reporting in interstitial HDR-BT, reporting vaginal doses to volumes of at least 2 cc is reliable and representative of the hottest dose to the vaginal mucosa. These hot spot volumes will occur near the surface of the needles or cylinder, and may not be contiguous but truly represent the hottest dose in complex interstitial dose distributions, while standard points based on cylinder position do not.
The clinical standard will continue to have clinicians reviewing brachytherapy treatment plans using TG43-based dose calculation. The 2 cc volume metric satisfies the conditions for accuracy and precision at all dose levels investigated across large cohort of patients. It should be reiterated that the TG43-based dose calculation is generally a slight overestimate of the MC-based dose calculation. To establish dose-effect relationships for the mucosa, reporting small volume metrics from TG43-based calculations will result in difficulty establishing the correlation with outcomes. If MC-based dose calculation becomes the clinical standard, smaller volume metrics may be reported.
Scenarios where dose evaluation occurs very close to a source are subject to great uncertainties due to extrapolation of TG43 data in short distances and uncertainties in the source model [19]. Other groups have observed large discrepancies of > 20% between MBDCAs and TG43-based treatment planning systems near 192Ir source [18, 24]. For GYN perineal template interstitial HDR-BT cases, the vaginal mucosa often contains many interstitial needles, and proximity of sources to vaginal dose calculation points are likely subject to high uncertainties.
Discrepancies in vaginal dose calculation in this work result in part from the removal of dose deposited inside the source from dose distributions in MC, which is observed in the dose distributions shown in Figure 2 and is further detailed in Section 2 of the Supplementary information. These discrepancies would occur regardless of MC or TG43 implementation, assuming that the MC removes intra-source doses while TG43 implementations do not. In the context of GYN interstitial HDR-BT, Desbiens et al. [17] observed that exclusion of non-biological material (such as the cylinder and air pockets) is important in both MC and TG43. The HDR source is also non-biological material that for interstitial HDR-BT can represent a considerable portion of volume on the order of DVH metric volume. The simulated 192Ir flexi-source has a volume of approximately 8 mm3: 13 source positions dwelling inside the vaginal contour accounts for 0.1 cm3. Larger volume metrics, such as D2cc are greater than the order of source volume in the vaginal mucosa, and are therefore more reliable. Removing the CTV from the vaginal contour further increases reliability of TG43-calculated vaginal dose, since the CTV should contain the highest-weighted dwell positions. Modeling the interstitial needle lumen and removing it from the patient volume may further impact vaginal dose calculation.
Dose calculation deviations between TG43-based treatment planning software have been shown to be 4-8% (one standard deviation) for DVH metrics between 0.1 cc and 2 cc [25]. Treatment planning systems differ in region-of-interest reconstruction and interpolation strategies, which cause DVH metric uncertainties [25-27], especially when these small volumes are situated in high-dose gradient regions [25, 27] where uncertainties over 10% can be observed [25]. However, small volume DVH uncertainties between TG43 treatment planning systems are comparable to inter-observer variability [25], and improve when CT slice thickness is reduced [27], as this facilitates more accurate catheter reconstruction and structure contouring. All these findings suggest that small volume dose evaluation near HDR sources is unreliable, which we explicitly investigated for vaginal dose assessment in the current study.
In this study, a 5 mm expansion of the vaginal cylinder was used as a surrogate for the vaginal mucosa contour, as several groups have done previously [9, 11, 12]. The true vaginal mucosa is a complex structure with variable depths and folds and can be contoured in CT and MRI. However, contouring the vaginal mucosa on a per-patient basis for the sake of dose reporting may not be practical, especially when vaginal doses are typically not considered a high-priority planning aim. Cylinder expansion offers a quick, robust, and practical alternative vaginal mucosa volume. In this study, we demonstrated the problem with small volume vaginal dose reporting below 2 cc for perineal template interstitial implants.
This study shows that modeling the HDR source and removing its volume from the patient geometry causes large discrepancies in small volume vaginal dose metrics between MC and TG43. Excluding non-biological material, such as source volumes from the vaginal contour, better resembles the clinical vaginal dose. When evaluating vaginal dose in GYN perineal template interstitial HDR-BT, it is recommended that clinics with TG43 dose calculation use vaginal dose metric volumes of at least 2 cc and withdraw the CTV volume from the vaginal contour. This removes the influence of source modeling and facilitates reliable calculation of the vaginal hot spot dose in TG43-based treatment planning systems compared with MC. Reliable vaginal dose metrics are essential if dose-effect relationships for vaginal toxicities are to be established.
Conclusions
For gynecologic perineal template HDR-BT procedures, the 2 cc volume is the smallest representative volume that reliably reports dose to the vaginal mucosa. The correlation between TG43 dose and MC-based dose calculation for D2cc of the vagina is very high and, on average, within 5%. At a minimum, D2cc should be reported for the normal vaginal mucosa for dose and outcome evaluation. Dose reporting using accurate metrics is critical to establish dose-effect relationships for brachytherapy procedures with high-doses due to the proximity of the radiation source to the vaginal mucosa.