Summary
The aim of the study was to explore the relationship between cardiac troponin I (cTnI) elevation and supraventricular ectopy (SVE) following percutaneous closure of secundum atrial septal defect (ASD) in adult patients. 600 consecutive patients with secundum ASD who underwent successful transcatheter defect closure were analyzed. Serum levels of cTnI were measured before and within 72 h of device implantation. 24-hour Holter monitoring was performed before the procedure, at 1 month, and at 6 months of follow-up. The collected data, along with the procedural parameters, were subjected to multiple forward stepwise regression analysis. A transient postprocedural increase of cTnI was detected in the majority of patients. A significant increase in the number of SVE per 24 h was noted 1 month after the procedure, and positively correlated with cTnI elevation. There was also a positive correlation between cTnI rise, procedure duration, fluoroscopy time, and device size. A significant increase of cTnI is noted frequently after transcatheter ASD closure and seems to predict exacerbation in SVE burden on short-term follow up. The independent risk factors of cTnI rise are prolonged procedure duration and larger device sizes.
Introduction
Atrial septal defect (ASD) is the most common congenital heart defect observed in adults [1, 2]. According to the current European Society of Cardiology guidelines, percutaneous ASD closure should be the therapy of choice when anatomical conditions are favorable and is now a routine clinical practice [1–4].
Heart rhythm disturbances are one of the most prominent symptoms of ASD. In addition, transcatheter treatment itself may also induce arrhythmias such as atrial fibrillation (AF) or supraventricular tachycardia (SVT) in the early follow-up [5–9].
Interventional ASD occlusion causes much lower myocardial damage than surgical closure. However, little is known about myocardial lesions associated with occluder implantation [10, 11].
Cardiac troponin I (cTnI) is a very specific and sensitive marker of myocardial injury. A significant increase in cTnI levels after transcatheter ASD closure has been reported in the literature [12, 13].
We found it of clinical interest to determine whether potential endothelial and myocardial damage resulting from atrial instrumentalization during ASD closure is associated with troponin release and to establish a link between this injury and arrhythmias ensuing directly after and over weeks following the procedure.
Aim
This study aimed to identify cTnI rise after percutaneous ASD closure, to determine its prognostic value, and to assess its relationship with supraventricular ectopy (SVE) in early follow-up.
Material and methods
The analysis included 600 consecutive patients who underwent transcatheter ASD closure. During the follow-up after the procedure patients were observed, the troponin level was serially determined and Holter ECG monitoring was performed. The prior diagnosis of ASD was based on clinical evaluation and transthoracic echocardiographic study (TTE). The final qualification for the procedure was based on transesophageal echocardiography (TEE). Eligibility criteria for percutaneous closure were: the presence of a single ostium secundum ASD located in the central portion of the interatrial septum, encircled by adequate rims (size of at least 5 mm – except for the aortic rim), a significant left-to-right shunt measured on right heart catheterization (ratio of total pulmonary blood flow to total systemic blood flow [Qp/Qs] > 1.5 : 1), or shunting with echocardiographic signs of right ventricular volume overload, coexisting impairment of physical capacity, and absence of pulmonary hypertension.
In all patients septal occluders were successfully implanted in a typical fashion under local anesthesia, with continuous TEE monitoring and fluoroscopic guidance.
Serum levels of cTnI were determined from serial venous blood samples which were taken at five intervals: before the procedure and subsequently at 8, 16, 24, and 72 h thereafter. The cTnI levels were measured with the Architect STAT Troponin I assay (Abbott Diagnostic Division, Abbott Park, Illinois, USA) with a minimum detectable cTnI concentration of < 0.01 (ng/ml). To identify a prognostically significant cTnI rise after ASD closure, we examined whether the overall cTnI release correlated with total procedure duration, fluoroscopy time, device size, defect dimensions, and the burden of SVE 1 month after the procedure. 24-hour Holter monitoring was performed in all patients before the procedure and after 1 and 6 months of follow-up. Holter ECG monitoring was performed using a 3-lead Del Mar 563 recorder and analyzed with Del Mar Avionics software, reporting the mean, minimum, and maximum heart rate (HR) and the presence of any significant conduction or rhythm abnormalities, including SVE and ventricular extrasystoles (VES).
The study was approved by the local academic Research Ethics Committee. Written informed consent had to be obtained from every patient before each medical intervention.
Statistical analysis
Concerning statistical analysis, quantitative data are presented in the form of mean, standard deviation, median, minimum, and maximum values. Qualitative data are presented as the number of observations (n) and % of the total. The Wilcoxon rank-sum test was used to compare the amount of SVE/24 h in the Holter ECG before and after ASD closure. In order to evaluate the correlation between the number of arrhythmias on Holter ECG 1 month after surgery and troponin levels 24 h after surgery, and between troponin levels 24 h after surgery and surgical parameters, Pearson correlation analysis was used, and the result is presented as the correlation coefficient (r) and p-value. The results of the correlation are presented in table form and as figures. Linear regression analysis was used to assess the relationship between troponin levels 24 h after the procedure and individual procedure parameters. Results are presented as OR, 95% CI and p-value. Multivariate regression analysis, including backward stepwise regression analysis, was performed to assess the relationship between troponin levels 24 h after surgery and surgical parameters. Results are presented as OR, 95% CI and p-value. In addition, an analysis of the coefficient of determination R2, extremely important in this type of analysis, was performed. A value of p < 0.05 was considered statistically significant. Data were analyzed using Statistica 13.1 PL package (Dell Software Inc., Round Rock, TX, USA).
Results
The analysis included 600 consecutive patients: 354 (55.67%) women, 246 (44.33%) men, with a mean age of 39.49 ±19.74, who underwent transcatheter ASD closure. The device was successfully implanted in all patients (procedure time 20.00 ±6.31 min, fluoroscopy time 6.62 ±2.44 min. Information on defect size and shunting and procedural data are summarized in Table I.
Table I
ASD and procedure data of patients who underwent ASD procedure
A significant increase in SVE number per 24 h was noted 1 month after the procedure (median 1021.00; min.–max. 11.00–29862.00) compared to baseline data (median 146.00; min.–max. 0–1865.00; p < 0.01). After 6 months the SVE number decreased (median 142.00; min.–max. 0–1865.00) as shown in Table II and Figure 1.
Table II
Change in SVE burden on 24-hour Holter monitoring and troponin level following percutaneous ASD closure
Parameter | Mean SD | Median | Minimum | Maximum |
---|---|---|---|---|
SVE/24 h: | ||||
Before ASD closure | 229.15; 266.33 | 146.00 | 0.00 | 1865.00 |
1 month after closure | 3689.62; 5289.25 | 1021.00 | 11.00 | 29862.00 |
6 months after closure | 221.76; 258.36 | 142.00 | 0.00 | 1865.00 |
Troponin level [ng/ml]: | ||||
Before ASD closure | 0.000714; 0.000456 | 0.000900 | 0.000000 | 0.001500 |
First day after closure** | 0.135871; 0.240257 | 0.029500 | 0.000000 | 1.000000 |
Second day after closure | 0.000900; 0.001739 | 0.000900 | 0.000000 | 0.024000 |
The mean cTnI level before ASD closure was 0.0009 ng/ml median (0–0.0015). 24 h after implantation the mean cTnI level was 0.0295 ng/ml (0–1.0000). Within 24 h of device implantation an increase in troponin levels exceeding 50% of the upper reference limit was observed in 61.7% of patients. After 72 h cTnI levels decreased and normalized in all the patients (Tables II and III). This substantial increase in cTnI level was neither associated with signs and symptoms of myocardial ischemia or infarction nor other serious clinical complications in any patient. There was, however, a statistically significant positive correlation between 24-hour SVE burden 1 month after the procedure and periprocedural cTnI increase (p < 0.05, r = 0.41) as shown in Table IV. In addition, the degree of cTnI rise was significantly correlated with the procedure time (p < 0.001), fluoroscopy time (p < 0.001), device size (p < 0.001) and ASD maximal diameter (p < 0.001). The correlation and regression results are presented in Figure 2 and Table IV.
Table III
Percentage of patients displaying a significant cTnI increase within 24 h of transcatheter ASD closure
Parameter | Baseline | 8 h | 16 h | 24 h | 72 h |
---|---|---|---|---|---|
cTnI beyond 50% of upper reference level | 0% | 20% | 45% | 61.7% | 0% |
cTnI within normal range | 100% | 80% | 55% | 38.3% | 100% |
Table IV
Correlation between SVE burden on 24 h Holter ECG 1 month after procedure and peak cTnI within 24 h [ng/ml], and between SVE burden on 24 h Holter ECG 1 month after procedure and procedure parameters
Figure 2
Graphs of the relationship between procedural parameters and peak cTnI within 24 h post-procedurally [ng/ml]. A – Graph of the relationship between fluoroscopy time [min] and peak cTnI within 24 h post-procedurally [ng/ml]. B – Graph of the relationship between ASD maximal diameter [mm] and peak cTnI within 24 h post-procedurally [ng/ml]. C – Graph of the relationship between total procedure time [min] and peak cTnI within 24 h post-procedurally [ng/ml]. D – Graph of the relationship between device size [mm] and peak cTnI within 24 h post-procedurally [ng/ml]
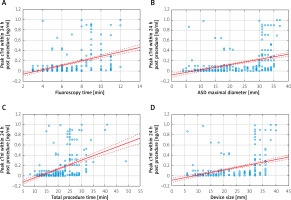
On single linear regression analysis the parameters sex, ASD maximal diameter, device size, total procedure time and total fluoroscopy time were associated with the observed cTnI increase during the first 24 h after the procedure (p < 0.05), as shown in Table V.
Table V
Linear regression analysis between patient demographics and procedure parameters and peak cTnI within 24 h after procedure [ng/ml]
On multiple linear regression analysis, of all the echocardiographic and procedural parameters, only total procedure time (p < 0.001; OR = 0.011; 95% CI: 0.004–0. 018) and total fluoroscopy time (p < 0.001; OR = 0.023; 95% CI: 0.007–0.040) were associated with the observed cTnI increase during the first 24 h after the procedure (Table VI). In backward stepwise regression, only the time of surgery influenced the cTnI value within 24 h after surgery.
Table VI
Multivariate linear regression analysis between patient demographics and procedure parameters and peak cTnI concentration at 24 h after surgery [ng/ml]. In backward stepwise regression, only the time of surgery influenced the cTnI value within 24 h after surgery
No significant relationships were found between rhythm disorders, as well as cTnI rise, in respect to size of the atria and right ventricle, age and degree of left-to-right shunting. Correlation analysis showed that the factors that significantly correlated with increased SVE 1 month after surgery were cTnI increase, surgery time, fluoroscopy time, ASD diameter and device size (p < 0.05 in all cases).
Discussion
Percutaneous ASD closure is nowadays a routine clinical practice [1–4]. Cardiac troponin is a very specific and sensitive marker of myocardial injury [14]. Surgical ASD closure leads to a transient increase in cTnI serum concentration [10, 14]. Interventional defect occlusion obviously causes lower myocardial damage than surgical closure; however, a temporary rise of cTnI concentration has also been reported after occluder implantation [12, 13]. ASD transcatheter closure induced myocardial injury, which is similar to other types of myocardial mechanical damage.
Some reports suggest that myocardium in children is more susceptible to trauma than in adults, based on higher area under the curve of cTnI levels after surgery at a younger age [15]. In our materials we observed a significant cTnI rise in adult patients regardless of the patient’s age. After interventional ASD occlusion, elevated cTnI levels for several hours may indicate some transient, reversible myocardial membrane instability linked to device placement.
Our results demonstrate a marked positive relation between the severity of myocardial injury, the size of the device used, and procedure duration. Thus, the longer the procedure and the larger the implant are, the greater is the myocardial damage. This may be associated with prolonged instrumentalization of the atria. It is not clear, however, whether troponin monitoring after percutaneous ASD closure could have a clinical application. In our material there was a significant positive correlation between periprocedural cTnI rise and SVE burden on 24-hour Holter monitoring 1 month following the procedure with a subsequent decrease at 6 months follow-up. In contrast, there were no apparent changes in the number of ventricular arrhythmias at the same time. Therefore, cTnI elevation after interventional ASD closure seems to predict a higher probability of temporary SVE exacerbation and could warrant a closer follow-up in selected patients. Hill et al., who analyzed Holter ECG recordings of 41 patients before and after percutaneous secundum ASD closure, drew similar conclusions. They observed a significant increase in supraventricular tachyarrhythmia without changes in VES burden [16].
Troponin release after defect closure may also be linked to technical aspects of the procedure itself. The invasive nature of the intervention, instrumentalization of the atria and device implantation may damage the wall of the heart chambers, provoke a cTnI rise and hence cause rhythm disturbances. Exacerbation of supraventricular arrhythmias after defect closure may be triggered by pathological automatism. Injury and scars from device implantation or replacement fibrosis may also provide a substrate for reentry. The dense fibrosis creates conduction block that can define borders of reentry circuits [9]. At the microscopic level, fibrosis separates myocyte bundles, forcing the excitation wave front to take a circuitous course through the bundles. In addition, conductivity between cells can be diminished. This uncoupling of myocyte bundles, and to some extent the myocytes within the bundles, slows conduction, although action potentials and ion channels in the myocytes can be relatively normal. Slow conduction and fibrous anatomic barriers set the stage for reentry [5, 9, 17, 18].
We observed a positive correlation between the cTnI rise in the first 24 h and fluoroscopy time. Fluoroscopy time may be regarded as an index of procedural complexity. Any challenging intervention is likely to prolong fluoroscopy time. The more time needed for appropriate device implantation, the higher the likelihood of traumatizing the atrial wall and septum, which in turn may induce cTnI release and arrhythmia [7, 18–23]. An additional positive correlation between device size and cTnI rise was also observed. The size of the device, similarly to fluoroscopy time, may predict difficulties during intervention. In contrast, a greater weight and size of the device may solely have an impact on atrial walls and provoke cTnI release and supraventricular arrhythmias [9, 20–23]. Percutaneous closure of ASD may result in reversible myocardial damage, which can be reduced by shortening the procedure as much as possible, and careful and attentive instrumentation in the lumen of the atria.
The periprocedural cTnI elevation may anticipate an increased number of supraventricular ectopic beats within the first 6 months after device implantation. More studies are needed to further clarify the exact role of postprocedural troponin measurement and periprocedural arrhythmias after 6 months of the postprocedural period in cardiovascular risk prediction in patients undergoing transcatheter ASD closure. Longer follow-up beyond 6–12 months is necessary to determine whether the increased number of arrhythmias persists longer after the transcatheter procedure.
Conclusions
A significant increase of cardiac troponin is observed frequently after transcatheter ASD closure and is not associated with signs and symptoms of myocardial infarction or other serious clinical complications. Percutaneous closure of ASD may result in reversible myocardial damage. The independent risk factors for cTnI rise are prolonged procedure duration and larger device sizes. The periprocedural cTnI elevation may anticipate an increased number of supraventricular ectopic beats within the first month after device implantation. More studies are needed to further clarify the exact role of postprocedural troponin measurement in cardiovascular risk prediction in patients undergoing transcatheter ASD closure.