Introduction
An invasion of Mycobacterium tuberculosis (Mtb) causes tuberculosis (TB), but in most people Mtb persists and causes a clinically insignificant infection known as latent tuberculosis infection (LTBI) [1, 2]. One quarter of the earth’s population consists of LTBI patients, and studies have also shown that approximately 5-10% of untreated LTBI patients would progress to active tuberculosis during their lifetime [3-5]. Thus, the large number of LTBI patients led to the global TB pandemic.
Due to the lack of a gold standard, diagnostic methods for LTBI patients rely on a positive tuberculin skin test (TST) and/or a blood-based interferon-gamma release assay (IGRA). But extensive research has shown that neither test is perfect, and a variety of immunocompromised people have low sensitivity [6-8]. Therefore, how to identify LTBI patients from the population and how to identify LTBI patients who will soon develop APTB patients is critical. The reason for our failure in these aspects lies in the lack of thorough research and understanding of the mechanisms of anti-TB immunity and immune abnormalities in LTBI patients.
NK cells are a crucial constituent of innate immunity but also have an adaptive immune effect, whose key features is to kill cells infected with Mtb [9-11]. Interferon γ (IFN-γ) produced by NK cells activates the antimicro-bial effect function of macrophages, which is critical for the control of Mtb. NK cells can also produce perforin to control the growth of Mtb. In addition, NK cells have been found in lung mature granulomatous lesions in patients infected with Mtb [12]. The level of CD56 expression provides a functional classification of human NK cells [13, 14]. CD56bright NK cells are less mature and regulate immune responses primarily by secreting cytokines. The CD56dim subgroup (> 90%) represents the majority of circulating NK cells which have a powerful killing function [15, 16]. However, it is regrettable that the functional changes of the two subpopulations of NK cells have not been widely studied in LTBI patients.
Here, therefore, the numbers and immunophenotypes of circulating NK cells and their two subpopulations in LTBI patients were described and compared with HCs and APTB patients. Our results provide a new basis for clinical formulation of immunization interventions.
Material and methods
Patients
Twenty-one LTBI patients, 25 APTB patients and 25 healthy age- and sex-matched subjects as HCs participated our research (Table 1) after obtaining complete ethi-cal approval and informed consent. The research protocol complies with the Declaration of Helsinki. As with most studies, enrolled LTBI patients had no symptoms of active tuberculosis and could not be clinically identified by bacteriology (antacid smear or gene X-PERT), imaging, etc., but their T-spot test was positive [17, 18]. All patients with APTB were confirmed by bacteriology.
Isolation of peripheral blood mononuclear cells (PBMCs)
One milliliter of diluted human peripheral blood was added to the top of Ficoll lymphocyte separation solution, and after centrifugation, the mononuclear cell layer was absorbed into the flow tube, washed and set aside.
Flow cytometry analysis
The selected antibodies, including FITC Anti-human CD3, APC/Cy7 Anti-human CD8, BV-510 Anti-human CD56, APC Anti-human granzyme A (GrA), PE Anti- human CXCR3, PE Anti-human granzyme B (GrB), BV421 Anti-human HLA-DR and BV-421 Anti-human Perforin (PFP), all came from BioLegend (San Diego, CA). Circulating mononuclear cells were extracted from all individuals, labeled with surface markers, then fixed with paraformaldehyde, and finally stained with intracellular markers. FACS Canto II (BD) was used to measure the percentage of cells. Gating strategies for flow cytometry could be found in Supplementary Material.
Methods for counting the total number of NK cells and their subpopulations
Patients in each group collected 2 ml of EDTA-anticoagulated whole blood, and leukocyte classification (neutrophils, eosinophils, basophils, monocytes and lymphocytes) was detected by an automatic blood cell analyzer (Sysmex F6709). The total number of NK cells was the number of lymphocytes multiplied by CD3– CD56+ %. Both the total number of CD3– CD56dim and the total number of CD3– CD56bright were the number of lymphocytes multiplied by the corresponding percentage.
Statistical analysis
GraphPad Prism software (v. 7.0a; GraphPad Software, La Jolla, CA) was used to analyze the obtained data. Variables subject to normal distribution were expressed by mean ± standard deviation, otherwise by interquartile interval and median. The t-test or Mann-Whitney U test was performed for comparison between the groups. For all tests, a bilateral p-value < 0.05 was considered significant (****p < 0.0001, ***p < 0.001, **p < 0.01 and *p < 0.05).
Results
The number of NK cells and their subsets in LTBI patients
Initially, we detected the number of NK cells and their subgroups in the peripheral blood of LTBI patients. The results showed that compared with HCs, the absolute number of NK cells in patients with LTBI was not significantly different, but it was higher than that in APTB patients (Fig. 1A), specifically manifested as an increase of CD56bright and CD56dim NK cells (Fig. 1B, C).
Activation status of NK cells and their subpopulations in LTBI patients
The frequencies of HLA-DR+ NK cells in LTBI patients did not differ significantly compared with HCs and APTB patients (Fig. 2A). However, further examination showed that the frequency of HLA-DR+ CD56bright NK cells in LTBI patients was significantly lower than that in HCs and APTB patients (Fig. 2B).
Expression of GRA, GRB and PFP in NK cells and their subpopulations in LTBI patients
Detection of expressed toxic particles revealed that the expression of GrA in NK cells of LTBI patients was not as high as in APTB patients (Fig. 3A), and the expression of GrB and PFP was not significantly different (Fig. 3B, C). Further detection showed that, compared with HCs, the intracellular GrB levels in CD56bright NK cells were as significantly elevated in LTBI patients as in APTB patients (Fig. 4B), while their intracellular GrA and PFP levels were both significantly lower than in APTB patients (Fig. 4A, C).
Fig. 3
GrA+/GrB+/PFP+ expressed in NK cells in LTBI patients. A-C) Cytoendocrine GrA, GrB and PFP levels of NK cells in the three groups. GrA+/GrB+/PFP+ percentages represent the frequency of cytoendocrine GrA, GrB and PFP in three types of cells, respectively
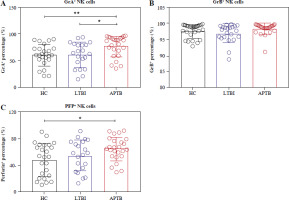
Fig. 4
GrA+/GrB+/PFP+ expressed in CD56bright and CD56dim NK cells in LTBI patients. A, D) Intracellular GrA expression levels of CD56bright cells and CD56dim NK cells in the three groups. B, E) Intracellular GrB expression levels of CD56bright cells and CD56dim NK cells in the three groups. C, F) Intracellular PFP expression levels of CD56bright cells and CD56dim NK cells in the three groups
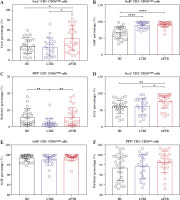
Expression of CXCR3 on NK cells and their subpopulations in LTBI patients
Compared with HCS, the frequencies of CXCR3+ NK cells, CXCR3+ CD56bright NK and CXCR3+ CD56dim NK cells were greatly reduced in both LTBI and APTB patients (Fig. 5).
Fig. 5
CXCR3 expressed on NK cells in LTBI patients. A-C) Flow cytograms display the expression of CXCR3 on the NK cells, CD56bright cells and CD56dim NK cells in the three groups, respectively. The quadrants indicate the frequency of CXCR3+ NK, CXCR3+ CD56bright or CXCR3+ CD56dim NK cells D) Expression of CXCR3 on three types of cells
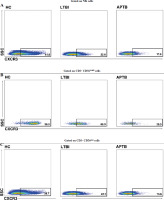
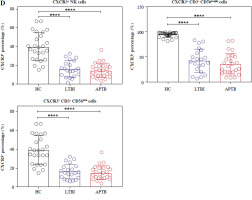
Discussion
Latent tuberculosis infection accelerates the global TB pandemic. The association between NK cells and resistance and susceptibility in APTB patients has been explored in a series of studies, but the phenotype of the two subpopulations of NK cells has not been widely discussed in LTBI patients. We hope that the in-depth research on NK cells in LTBI patients will introduce new ideas for LTBI to be conquered.
Initially, we were interested in the absolute number of NK cells. It was found that there was no change in the number of NK cells in LTBI patients and HCs, both of which were higher than those with APTB patients. This was the same as the findings of Nurhan Albayrak et al. [19]. It indicated that LTBI patients still had ample NK cells to fight against Mtb and maintain the immune balance of the body, so there had been no clinically significant symptoms.
Meanwhile, the frequency of HLA-DR+ NK cells in LTBI patients was found not to differ significantly compared with that in HCs and APTB patients. Research has shown that HLA-DR+ CD56dim cells possess higher IFN-γ secretion and higher degranulation ability. Moreover, in vitro studies discovered that HLA-DR+ NK cells could mediate the production of higher levels of cytokines in autologous CD4+ T cells induced by Mtb [20]. This implied that the link between NK cells and adaptive immunity was strengthened by HLA-DR expression on NK cells. Interestingly, our results demonstrated that the frequency of HLA DR+ CD56bright NK cells in LTBI patients was not as high as that in HCs and APTB patients.
Then the toxic status of NK cells was detected, and only LTBI patients had lower intracellular GrA expression than APTB patients. Surprisingly, however, our further exa-mination of two subpopulations of NK cells for intracellular granzyme and perforin found that LTBI patients had higher levels of GrB and lower levels of PFP in their CD56bright cells compared with HCs. This meant that the ratio of GrB+ CD3– CD56bright and PFP+ CD3– CD56bright NK cells could be used to effectively distinguish between HCs and LTBI patients. Also, previous studies had considered that after a decrease in PFP, GrB could not enter target cells or only a small amount of GrB enters target cells through endocytosis [21]. In LTBI patients, the decrease of CD56bright intracellular PFP and the increase of GrB made it not produce a continuous killing effect, and avoided excessive inflammation to maintain the immune balance between Mtb and the host organism. However, with a high load of Mtb in APTB patients, CD56bright cells need to generate high levels of PFP and GrB to lyse Mtb.
Due to the limitation of objective conditions, we could not obtain cells from the infected site. Consequently, we only examined the migration ability of NK cells. We discovered that the frequency of NK cells carrying CXCR3 was significantly lower in circulating of LTBI patients compared with HCs, as evidenced by lower frequencies of CD56bright and CD56dim NK cells carrying CXCR3. It was found in our previous research that the concentration of CXCL10 and CXCL11, ligands of CXCR3, in pleural fluid was higher than that in the circulation [22], forming a concentration gradient, which also triggered the migration of CXCR3+ NK cells in circulation of LTBI patients to the site of lung infection.
In conclusion, our view is that circulating CD56bright NK cells have a prominent role in maintaining immune balance in LTBI patients. Moreover, an elevated frequency of GrB+ CD56bright NK cells and a reduced frequency of PFP+ CD56bright NK cells were effective in differentiating LTBI patients from HCs.