Purpose
According to the statistics, about 4,824,700 new cancer cases and 2,574,200 new cancer deaths occurred in China in 2022. Tumors of the lung, colon-rectum, thyroid, liver, and stomach were the top five cancer types, accounting for 57.42% of new cancer cases[1]. Presently, malignant tumor incidence and mortality rates are rising worldwide [2], with advanced malignant tumors ranking second to cardiovascular diseases in terms of mortality [3], posing clinical challenges for oncologists. Prolonging and enhancing lives of patients with advanced malignant tumors are the shared goals of patients, their families, and healthcare providers.
Currently, systemic therapy combined with local interventions is recognizable in treating advanced malignant tumors, employing various modalities, such as radiotherapy, interventional therapy, radio-frequency ablation, and deep hyperthermia. Even though initially regarded as a remedial approach, in recent years, studies have highlighted the efficacy of radiation therapy as an adjunct before, during, and after surgery in terms of reducing local tumor recurrence rates [4, 5]. In specific tumor types, radiation therapy can achieve radical cure. The utilization of iodine-125 (125I) radioactive particles as a local treatment method effectively controls tumor growth through intervention, emitting 27.4-31.4 keV X-ray and 35.5 keV γ-ray, with a half-life of 59.4 days and minimal impact on adjacent normal tissues [6]. Therefore, particle implantation, as a method of brachytherapy, is applied in ineffective or recurrent lesions after chemoradiotherapy for better local control [7, 8] in liver and lung cancers as well as bone and pelvic malignancies. In 1985, deep hyperthermia was approved by the Food and Drug Administration as the fifth major tumor treatment method after surgery, radiation therapy, chemotherapy, and biological therapy. It exploits temperature tolerance differences between normal and tumor cells to accumulate heat at the tumor site, effectively destroying malignant cells [9] while minimizing toxic side effects experienced with radiotherapy and chemotherapy [10, 11].
This retrospective study included data analysis of 60 patients, and the clinical efficacy and adverse reactions of 125I radioactive particle implantation combined with deep hyperthermia treatment were evaluated and summarized.
Material and methods
Patient clinical data and general procedures
Clinical data of 60 patients with malignant tumors who visited the Interventional Department of Zhongshan Hospital affiliated with Dalian University, from January 2021 to January 2023 were employed. The clinical efficacy and adverse reactions of 125I radioactive particle implantation combined with deep hyperthermia treatment were analyzed and summarized.
This cohort included 25 liver malignant tumor cases, 8 colorectal cancers, 7 pancreatic cancers, 6 cases of bile duct cancer, 3 tumors in the urinary system, 3 skin malignant tumors, 2 cases of lung cancer, 2 of gastric cancer, 1 case of ovarian cancer, 1 of breast cancer, 1 of cervical cancer, and 1 of endometrial cancer. Of these, 38 patients were males and 22 were females, with age ranging from 26 to 82 years (average age, 64.65 ±11.42 years). Disease staging revealed 19 stage III cases and 41 stage IV patients. The median number of implanted particles was 35 (range, 11-132), and the median dose activity was 0.6 (range, 0.56-0.8) mCi. All patients were confirmed through pathological diagnosis, and they were experiencing varying degree of cancerous pain. Pre-operative Karnofsky performance status (KPS) score exceeded 70 points. Computed tomography (CT)-guided 125I radioactive particles were implanted into the lesion, followed by deep hyperthermia treatment post-surgery. Monthly evaluations using CT or magnetic resonance imaging (MRI) were employed to assess clinical efficacy. This study was approved by the Ethics Committee of Zhongshan Hospital affiliated Dalian University, with informed consent obtained from all patients and their family members. The patient clinical data and procedures are shown in Table 1.
Table 1
General information of the patients
[i] Malignancies involved in this study, such as colorectal cancer, pancreatic cancer, bile duct cancer, urinary system tumor, skin malignant tumor, lung cancer, gastric cancer, ovarian cancer, breast cancer, cervical cancer, and endometrial cancer, all used American Joint Committee on Cancer (AJCC) classification. According to the actual situation of liver malignant tumor patients in China, China liver cancer staging (CNLC) was employed for classification.
Indication and contraindications
Inclusion criteria were as follows: 1) Pathologically diagnosed malignant tumors; 2) Age ≥ 18 years ≤ 85 years, with informed consent obtained from the patient and family as well as signed informed consent form for treatment and data analysis; 3) Presence of at least one measurable lesion according to solid tumor response criteria [12]; 4) KPS score ≥ 70 points, with an expected survival period exceeding 6 months [13]; 5) Child-Pugh A-B liver function grading; 6) No contraindications for particle implantation therapy.
Exclusion criteria were as follows: 1) Previous particle implantation therapy; 2) Utilization of other treatments, such as radiotherapy and chemotherapy after implantation and hyperthermia treatment; 3) Pregnancy or menstruation on the day of implantation and hyperthermia treatment; 4) Contraindications to particle implantation therapy; 5) Severe dysfunction or failure of organs, such as heart and liver; 6) Hemorrhagic diseases and coagulation disorders; 7) Fever exceeding 38°C, and body temperature regulation disorders; 8) Lack of regular monthly follow-up examinations post-surgery, and interruption of treatment; 9) Presence of a pacemaker.
Instruments and equipment
Radioactive 125I particles were provided by Beijing Zhibo High-Tech Biotechnology Co., Ltd., with radioactivity of 0.56-0.80 mCi, diameter of 0.8 mm, single particle length of 4.5 mm, half-life of 59.6 days, tissue penetration capacity of 1.7 cm, and nickel titanium alloy shell. Equipment employed included: Beijing Tianhang Kelin particle implantation plan system, treatment planning system (TPS, Beijing Fei Tian Technique Industries Inc., Beijing, China), particle implantation positioning navigation system, Mick particle implantation gun, Toshiba CT simulation positioning device, intelligent deep-focused beam heat therapy system (high frequency therapy machine), and WE2102-A microwave therapy unit.
Treatment methods
125I radioactive particle implantation
Patients were immobilized with a vacuum pad in a suitable position, and underwent a CT scan before 125I implantation. Using pre-operative CT or MRI data as well as other relevant imaging, tumor location, size, number of lesions, and other conditions were determined. The position lines were marked on the patient skin according to tumor’s central location. CT simulation images were transferred into treatment planning system (TPS), which was used to determine the tumor target dose, number of implanted particles, and placement location. Both GTV and organs at risks (OARs) were defined and delineated. Dose algorithm was calculated according to the official report of American Association of Medical Physicians [14, 15], and imaging indicated the tumor range as gross tumor target area (GTV). After a 5 mm expansion, clinical target area (CTV) was generated, and dual prescription doses of GTV and CTV were administered separately [16]. It was necessary for the plan to meet the dual prescription dose requirements while delineating OARs[17]. The planned pre-operative GTV D90 was 140 (range, 110-170) Gy, CTV dose was 100 (range, 70-130) Gy, median particle number was 35 (range, 11-132), and median dose activity was 0.6 (range, 0.56, 0.8) mCi. Intravenous anesthesia was applied in all patients for pain elimination and efficient procedure. Routine electrocardiogram monitoring was performed during surgery, with 125I particles placed according to the pre-operative plan guided by CT scanning positioning. Due to different conditions of the tumor, the radioactive particle implantation was mainly divided into CT-guided free-hand implantation technique, CT-guided and 3D-printed template-assisted technique, and CT-guided and 3D-printed non-coplanar template (3D-PNCT)-assisted technique. CT scanning was performed again on the 3rd day after the surgery, reviewed to evaluate surgical quality, and the radiation cold area was supplemented with implanted particles to meet radiation dose requirements of the entire target area. Additionally, CT scan confirmed particle distribution, with possible particle displacement, blood, fluid, and gas accumulation in the surgical area, and to verify post-operative dose of particles. The particle area was well-distributed, no related complications were detected, and deep hyperthermia was started. If any complication during and after the surgery were observed, corresponding treatment would be provided. In case of unstable vital signs, patient would be admitted to the intensive care unit for observation.
Deep hyperthermia
Deep hyperthermia treatment was started using an intelligent deep beam wave hyperthermia system (high frequency treatment device) and WE2102-A microwave treatment unit, as shown in Figure 1. The particle implantation site served as the temperature measurement point, with heating temperature maintained at 41-43°C for 30 min by a 12-channel real-time temperature measurement system, as shown in Figures 2 and 3. The interval between deep hyperthermia treatments was 48-72 hours. Within 6 months after particle implantation, deep hyper therapy was performed twice a week for at least 6 months. Patient vital signs, body temperature, and local skin conditions were monitored. Overheating was avoided, with heat was applied according to patient’s tolerance. Adjustments to output power or treatment cessation were implemented if abnormalities occurred, followed by corresponding symptomatic treatment. In case of severe symptoms, hyperthermia would be terminated. However, there were no severe symptoms requiring termination of hyperthermia in the patients involved in this study.
Fig. 1
Hyperthermia devices. A) Intelligent deep beam wave hyperthermia system (high frequency treatment machine); B) WE2102 – A microwave treatment unit
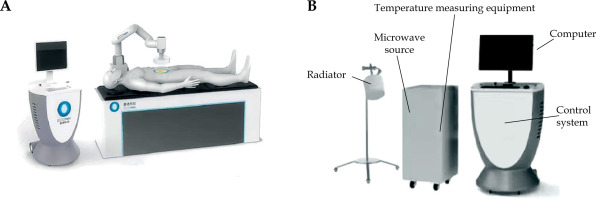
Evaluation indicators
Clinical efficacy evaluation
After particle implantation surgery, clinical efficacy was evaluated monthly through CT or MRI examinations. Evaluations allied with revised solid tumor response standards (RECIST, version 1.1) [12] were categorized into four levels: 1) CR (complete response) – full enhancement or disappearance of arterial phase target lesions; 2) PR (partial response) – total reduction in the diameter of target lesion (enhanced arterial phase) ≥ 30%; 3) SD (stable disease), diameter of the target lesion did not decrease to PR, nor did it increase to disease progression (PD); 4) PD, diameter of the target lesion increased by 20% compared with baseline value, or new lesions appeared. Additionally, objective response rate (ORR) was calculated as ORR = (CR + PR)/total number of cases × 100%, and disease control rate was determined as DCR = (CR + PR + SD)/total number of cases × 100%.
Quality of life evaluation
Employing a survey questionnaire, the present study evaluated patient quality of life using a core quality of life scale, i.e., the European Organization for Research and Treatment of Cancer quality of life questionnaire-C30 (EORTC QLQ-C30, version 3.0), a widely used global standard launched by the European Cancer Research and Treatment Organization in 1993 [17]. This scale, extensively applied in various advanced malignant tumors, such as lung cancer [18], breast cancer [19], cervical cancer [20], and other diseases, facilitates the assessment of patient’s quality of life. Using the EORTC QLQ-C30 (v. 3.0) scale, patient’s quality of life before and after 1-6 months of combined treatment was evaluated. The scale includes 30 items, and examines five functional areas, including physical, role, emotional, cognitive, and social functions. Rough score for each field was derived by summing the scores of included items and dividing by their number. Linear transformation was employed to convert RS into a standardized score (SS) within a range of 0-100. For functional areas, a higher score indicated an improved functional condition and quality of life.
Efficacy evaluation of cancer pain
Patient’s pain was evaluated using a numerical rating scale (NRS) [21], ranging from 0 (painless) to 10 (most painful), with 1-3 indicating mild pain, 4-6 moderate pain, and 7-10 signifying severe pain. Pain treatment efficacy categories included CR (painless), PR (significant pain reduction with undisturbed sleep and normal life), MR (pain somewhat alleviated, with significant remaining pain and disturbed sleep), and NR (no reduction in pain). The overall pain relief rate was calculated as (CR + PR)/total number of cases × 100%. Pain efficacy was observed from particle implantation surgery commencement to 6 months post-surgery.
Adverse reactions and complications
Adverse reactions and complications during the treatment period were evaluated using an acute radiation injury score from the Radiation Therapy Oncology Collaborative Group/European Organization for Cancer Treatment and Research [22]. During the treatment, the patients were carefully instructed to indicate any unpleasant feeling, such as a burning sensation, feeling of pressure, and any pain [23]. Level 1 damage included radiation-induced effects, such as slight skin atrophy, pigmentation, decreased white blood cell content (3.0-4.0) × 109/l, neutrophils (1.5-9.0) × 109/l, etc. Level 2 damage encompassed more severe radiation-induced effects, including flaky skin atrophy, moderate telangiectasia, complete hair loss, and decreased white blood cell content (2.0-3.0) × 109/l and neutrophils (1.0-1.5) × 109/l, etc.
Statistical methods
SPSS v. 23.0 was used to conduct statistical analyses, with normally distributed data presented as means ± standard deviations, and general information were expressed through frequency (n) and percentage (%) values. Pre-operative and post-operative indicators were compared using paired t tests and rank sum tests. P < 0.05 was considered statistically significant. Graphs were generated using GraphPad Prism v. 8.0.1.
Results
Statistical analysis of ORR and DCR
The clinical efficacy was evaluated monthly after particle implantation surgery using CT or MRI examinations. The clinical efficacy, ORR, and DCR of 60 patients at 1, 2, 3, 4, 5, and 6 months after treatment are shown in Table 2.
Quality of life and changes in pain
Quality of life and pain changes were assessed using EORTC QLQ-C30 (v. 3.0) and NRS scores before and at 1-6 months after treatment. Scores for the five functional areas of EORTC QLQ-C30 (v. 3.0) are shown in Tables 3 and 4. Significant differences were observed in physical function (PF), role function (RF), emotional function (EF), cognitive function (CF), and social function (SF) scores at 2, 3, 4, 5, and 6 months after combined treatment, compared with pre-operative scores (p < 0.05, Figure 4).
Table 3
EORTC QLQ-C30 (v. 3.0) rough score
Table 4
EORTC QLQ-C30 (v. 3.0) standardized score
Fig. 4
Functional domain scores before and after combined therapy. A) Physical function; B) Role function; C) Emotional function; D) Cognitive function; E) Social function. *p < 0.05, **p < 0.01, ***p < 0.001
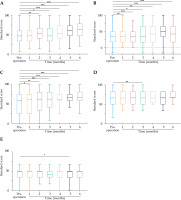
The NRS score decreased from 7.6 ±0.8 before treatment to 6.9 ±1.2, 5.8 ±1.1, 4.6 ±1.1, 5.1 ±1.0, 4.9 ±1.1, and 5.0 ±1.1 after 1 month, 2 months, 3 months, 4 months, 5 months, and 6 months of combined therapy, respectively (Table 5). Compared with before treatment evaluation, the patients’ pain scores decreased significantly after 1 month of combination treatment (p < 0.05, Figure 5). Among the 60 patients, 11 (18.33%) had ineffective pain relief (NR) and 49 (81.67%) had effective pain relief (PR + MR), but no patients achieved complete post-treatment pain relief (CR). The overall pain relief rate (CR + PR) was 37 (61.67%).
Safety evaluation
Deep hyperthermia, a painless and non-invasive treatment, caused no related adverse reactions during the treatment process. However, adverse reactions associated with 125I radioactive particle implantation included five cases of perioperative bleeding, eight of local mild inflammatory reactions after particle implantation, four of leukopenia, and two of particle implantation-related radiation damage (Table 6). All adverse events showed recovery after targeted supportive treatments. No patients exhibited symptoms of radiation damage to adjacent organs or other complications, such as particle displacement. Also, no treatment-related deaths were recorded.
Discussion
The global rise in malignant tumor incidence necessitates effective interventions [24]. Advanced malignant tumors, often infiltrating vital organs, blood vessels, and neural structures, result in diverse clinical symptoms causing pain and profoundly affecting patients’ quality of life. This scenario poses challenges for precise radiation therapy. The rapid development of 125I radioactive particle implantation technology has expanded its application across various solid malignancies. For example, Mo et al. [25] used 125I radioactive particles to treat patients with metastatic soft tissue sarcoma after first-line chemotherapy failure, with local control rates of 71.1% and 62.2% achieved at 3 and 6 months after surgery, respectively. Zhongmin et al. [26] employed CT-guided 125I radioactive seed implantation to treat patients with unresectable pancreatic cancer, reaching a disease control rate of 90.3% at 2 months post-treatment. As reported by Dai et al. [27], at 6 months after implantation, ORR was 55.4% and DCR was 98.2% in non-small cell lung cancer cases treated with 125I radioactive particle implantation. Moreover, Merten et al. [28] applied regional deep hyperthermia combined with other treatment methods, which effectively improved local control. These results align with the findings of the present study. After 1, 2, 3, 4, 5, and 6 months of treatment with 125I radioactive particles combined with hyperthermia, disease control rates were 80%, 91.67%, 83.33%, 71.67%, 78.33%, and 81.67%, respectively. This emphasizes the clinical efficacy of 125I radioactive particles combined with hyperthermia in local tumor control, leveraging the synergistic benefits of comprehensive treatment for enhanced patient outcomes.
Li et al. [29] reported that CT-guided 125I radioactive particle implantation effectively alleviated patients’ pain and improved their quality of life. Among the 60 patients in the current study, 17 (28.33%) showed ineffective pain relief, 43 (71.67%) experienced pain relief, and 9 (15%) presented significant pain relief. Given that tumors are most sensitive to radiation during the late stage of DNA synthesis and mitosis during the growth process, high tumor control rates and faster pain relief are achieved after 125I radioactive particle implantation [30]. In patients with severe pain (NRS, 7-10 points), pain relief was more pronounced after 125I radioactive particle implantation [31]. Moreover, such implantation combined with hyperthermia not only decreases lesion site nerve excitability, but also reduces adjacent muscle tension to achieve analgesic effects. Furthermore, hyperthermia, by increasing local blood flow and modulating inflammatory factors, such as prostaglandin, endothelin, bradykinin, IL-1, and IL-6, further contributes to pain alleviation. The present study underscores the synergistic pain-alleviating effects of 125I radioactive particle implantation with deep hyperthermia.
Late-stage cancer patients often experience debilitating symptoms, such as weight loss and cachexia, which markedly affect their daily activities as well as diminishing quality of life through heavy physical pain and psychological pressure. Assessing and enhancing the quality of life for these patients has become integral part in clinical efficacy evaluation. Indeed, quality of life assessment is an independent indicator in clinical efficacy evaluation. However, since such assessment is highly subjective, EORTC QLQ-C30 (v. 3.0) scores were obtained for patients through a questionnaire survey, briefly explained by the same physician, and then completed independently by the patient. Following seven evaluations, patient quality of life scores after combined treatment showed an upward trend, with significant improvements in PF, RF, and EF scores as well as CF. Following 125I radioactive particle implantation, some patients with skin-based malignant tumors and superficial malignant tumors develop atrophy and experience legion rupture. However, deep hyperthermia after surgery not only reduces swelling symptoms around such wounds, but also promotes wound healing and improves patient quality of life. To explain this phenomenon, additional theoretical support is required, which can be derived from multicenter and large-sample clinical trials and animal experiments.
Both 125I radioactive particle implantation therapy and hyperthermia cause only mild side effects and offer favorable safety profiles [32]. The present study is in line with Zhang et al. [33] who found that 125I radioactive particle implantation in pelvic malignant tumor treatment reduced tumor burden with only mild adverse reaction. In addition, a phase II study of Schem et al. [34] involving pre-operative radiotherapy and chemotherapy combined with regional hyperthermia for the treatment of locally advanced recurrent rectal adenocarcinoma, revealed no increase in treatment-related long-term side effects. Hyperthermia controls the local environmental temperature at 41-43°C. Under this condition, normal tissues maintain their self-healing function, whereas DNA and protein synthesis are negatively affected in tumor cells [35], promoting tumor cell degeneration and necrosis without involving normal tissue cell metabolism. Notably, no adverse reactions above level 2 were observed in the present study. Moreover, the post-operative adverse reactions in all patients improved after targeted supportive treatments, and no treatment-related deaths were recorded. This favorable safety profile, combined with the effective outcomes, underscores the safety and efficacy of 125I radioactive particle implantation with hyperthermia as a combination therapy for malignant tumors.
In conclusion, 125I radioactive particle implantation, coupled with deep hyperthermia, presents a minimally invasive and well-tolerated treatment for advanced tumors. For inoperable stage III and IV advanced malignant tumors, this combined approach exhibits positive therapeutic effects, with robust short-term and long-term clinical efficacy, enhancing patient quality of life and alleviating local pain. However, the optimal timing for 125I radioactive particle implantation to control tumor progression and mitigate pain symptoms due to peripheral nerve invasion as well as the optimal duration of post-operative deep hyperthermia course for maximized clinical efficacy, remain as the areas for further investigation. Large-scale, multicenter studies, and potential inclusion of diagnostic and treatment norms, are crucial steps towards establishing a safer and more effective combination therapy for patients with advanced malignant tumors.