Purpose
Over the last decade, the rise in the length of survival for cancer patients has led to an increased incidence of brain metastases. Brain metastases have now been shown to occur in up to 40% of cancer patients and are the most common cause of intracranial tumors [1]. Without treatment, the prognosis is grim, with survival of only 1-2 months [2]. Surgical resection of the metastases has been shown to increase overall survival [3]. Resection, however, often requires adjuvant irradiation in the form of stereotactic radiosurgery (SRS) or whole-brain radiotherapy (WBRT) in order to prevent local recurrence. Due to WBRT’s quality of life concerns, its late toxicity profile and the fact that it offers no overall survival benefit compared to more local therapy, recent focus has shifted towards the potential of local post-resection options, such as SRS and intra-operative brachytherapy [4,5,6,7]. Brachytherapy, in particular, has received considerable interest as it offers some advantages even over SRS, namely its absence of a long-time frame that can allow tumor cell repopulation [4,8]. A recent study that our group has published has shown that surgical resection and intra-operative brachytherapy is more cost-effective compared to surgical resection and SRS in the treatment of brain metastasis [9]. Additionally, it is known that SRS does not achieve optimal local control for large (greater > 3 cm) and irregularly-shaped cavities [9].
Intra-operative brachytherapy implants have been utilized for the prevention of local recurrence in cases of brain metastases. Brachytherapy has historically utilized the iodine-125 (125I) radioisotope for both permanent and temporary seed implants [10]. The commonly used radioisotope 125I has a T1/2 of 59.4 days and has an average energy of 28.4 keV. These physical properties make 125I convenient for storage, with limited shielding. The clinical results have revealed that although 125I has been shown to confer local control comparable to that of post-operative SRS, the high rates of radiation necrosis (RN) associated with 125I brachytherapy have been criticized [11].
Cesium-131 (131Cs) is a relatively novel radioisotope, the Food and Drug Administration cleared in 2003, which presents both physical and radio-biological advantages when compared to 125I brachytherapy. 131Cs has a shorter T1/2 of 9.69 days compared to 59.4 days for 125I. 131Cs is more likely to be biologically efficient for the destruction of tumor cells, as the initial dose rate from 131Cs (23.9 cGy/h) is 4 times higher than that of 125I (5.8 cGy/h) [9,10]. Our institution was the first to report the results of a prospective phase I/II trial demonstrating the effective use of intra-operative application of 131Cs brachytherapy for patients with newly diagnosed brain metastases [4]. In that study, we reported a 0% incidence of clinical RN. This contrasts with the results reported by many studies that used permanent 125I seeds, which has been associated with an average RN rate of 14.4% (range, 0-26%) [12]. In the present study, we investigated the dosimetric profile of 131Cs brachytherapy compared to that of 125I in order to understand this disparity in RN rates.
Material and methods
Patient characteristics
There were 24 patients in total, 14 females and 10 males, with a median age of 65 years (range, 45-84 years). The brain metastases were located in the frontal (10), parietal (7), cerebellar (4), occipital (2), and temporal (1) regions. The histology from the metastases was lung (16), breast (2), kidney (2), melanoma (2), colon (1), and cervix (1).
Implantation procedure
Between 2010 and 2015, following Institutional Review Board approval, 24 patients were enrolled on a prospective trial at the New York Presbyterian/Weill Cornell Medical Center, and underwent neurosurgical resection and intra-operative 131Cs brachytherapy for newly diagnosed brain metastases. The metastases were detected in each patient using a magnetic resonance imaging (MRI) or computerized tomography (CT) scan. As described previously [4], directly after resection, 131Cs stranded seeds (IsoRay, Inc., Richland, WA, USA) with an activity of 3-5 mCi were implanted, with a planned dose of 80 Gy to a 5 mm depth from the surface of the resection cavity. At the time of resection, the seeds were secured with Surgicel® (Ethicon, Inc., a Johnson & Johnson Company, Somerville, NJ, USA) to prevent seed migration and alteration of dosimetry. Lastly, Tisseel® (Baxter International, Inc., Deerfield, IL, USA), a fibrin glue, was also placed within the cavity to limit cavity shrinkage and further prevent seed dislodgement. Post-operative CT scans (GE LightSpeed averaging 36.85 mGy) were performed within 48 hours after surgery to determine dose distribution. Follow-up physical evaluation and MRIs were performed every 2 months thereafter. For the simulated 125I isotope implants, the prescribed dose was 80 Gy as well. The exact implant scheme for 131Cs was used for the simulated 125I cases. Finally, RN was detected on follow-up MRIs and confirmed by a combination of clinical judgement and further imagine including perfusion, spectroscopy, and positron emission tomography (PET) scans.
Dosimetric comparison of 131Cs vs. 125I
Dosimetric comparison was carried out for all included 24 patients. Post-operative dosimetry plans were generated using BrachyVision (Varian Medical Systems, Palo Alto, CA, USA) for 131Cs seed model 131Cs Rev 2, and the same CT data set was re-planned with 125I seeds using model No 6711 (TG43). The mean air kerma strength for the 131Cs and 125I seeds was 2.4 U (3.768 mCi) and 0.6985 U (0.55 mCi), respectively. The dose volume histogram (DVH) was generated and from it, the volume of brain tissue (cc) exposed to radiation at the 100%, 90%, 80%, and 50% isodose lines were compared for both radioisotopes. From the DVH, the D100, D90, D80, and D50 were obtained as well. Lastly, the homogeneity and conformity indices were calculated for each individual patient. The equations used for HI and CI were as follows:
where D100 – volume enclosed by prescribed dose rate and D150 – volume enclosed by 1.5 times prescribed dose rate.
where TV – total volume receiving prescription dose and CTV equals clinical target volume.
The different mean values between 131Cs and 125I were compared using the Wilcoxon rank-rum test.
Results
Dosimetric comparison of 131Cs vs. 125I
The median volume of resected tumor based on pre-operative MRI was 10.31 cc (range, 1.77-87.11 cc), intra-operative measurements of the cavity revealed a median volume of 3.13 cc (range, 1.00-17.00 cc), and median number of seeds employed was 12 (range, 4-35 seeds). The clinical data from these patients resulted in 100% local control, with median overall survival of 9.9 months, and 0% incidence of RN. Table 1 shows the mean volume of brain tissue exposed to radiation for each of the four isodose lines from both 131Cs and 125I. At the 100% isodose line, the mean volume of brain tissue exposed was 15.44 cc (range, 2.50-52.78 cc) and 9.84 cc (range, 1.22-32.87 cc) for 125I and 131Cs, respectively (p < 0.001). At the 90% isodose line, the mean volume exposed was 17.51 cc (range, 2.90-59.05 cc) and 11.31 cc (range, 1.47-37.59 cc) for 125I and 131Cs, respectively (p < 0.001). At the 80% isodose line, the mean volume exposed was 19.96 cc (range, 3.30-67.20 cc) and 13.61 cc (range, 1.73-43.4 cc) for 125I and 131Cs, respectively (p < 0.001). At the 50% isodose line, the mean volume exposed was 33.40 cc (range, 6.30-109.70 cc) and 23.38 cc (range, 3.39-76.14 cc) for 125I and 131Cs, respectively (p < 0.001). Consistently, for each isodose line, the mean volume of brain tissue exposed to radiation was significantly higher (on average, 52.0% higher) for 125I than for 131Cs. Figure 1 displays three isodose lines for both 125I and 131Cs seeds and superimposes them, allowing the clear visualization of the contrasting volumes of brain tissue exposed to radiation.
Table 1
Mean volume of brain tissue exposed to radiation doses at different isodose lines by 131Cs and 125I isotopes
Isodose line | Mean volume of brain tissue (cc)131Cs | Mean volume of brain tissue (cc)125I | p-value |
---|---|---|---|
100% | 9.84 | 15.44 | < 0.001 |
90% | 11.31 | 17.51 | < 0.001 |
80% | 13.61 | 19.96 | < 0.001 |
50% | 23.38 | 33.40 | < 0.001 |
Fig. 1
Axial images of a patient who underwent neurosurgical resection and intra-operative brachytherapy demonstrating volume of brain tissue exposed to radiation across multiple isodose lines for each radioisotope and superimposed comparison between 131Cs and 125I isodose lines: A) plan using 125I, B) plan using 131Cs, C) 120 Gy isodose line, D) 80 Gy isodose line, E) 40 Gyisodose line
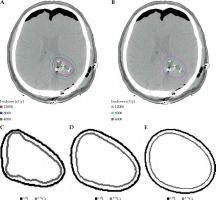
As Table 2 shows, the D100 was 64.96 Gy (range, 46.46-10.90 Gy) and 43.99 Gy (range, 26.19-74.09 Gy) for 125I and 131Cs, respectively (p < 0.001). The D90 was 119.02 Gy (range, 73.44-174.36 Gy) and 78.64 Gy (range, 40.31-136.36 Gy) for 125I and 131Cs, respectively (p < 0.001). The D80 was 141.78 Gy (range, 84.02-203.02 Gy) and 93.09 Gy (range, 45.80-161.16 Gy) for 125I and 131Cs, respectively (p < 0.001). The D50 was 206.17 Gy (range, 113.69-289.32 Gy) and 134.79 Gy (range, 61.85-228.86 Gy) for 125I and 131Cs, respectively (p < 0.001). At each percent volume of the CTV, the dose delivered by 125I was significantly higher (on average, 51.0% higher) than for 131Cs.
Table 2
Absolute doses of radiation delivered to certain percent volume of CTV by 131Cs and 125I isotopes
Percent volume of CTV | Dose of radiation (Gy) 131Cs | Dose of radiation (Gy) 125I | p-value |
---|---|---|---|
100% (D100) | 43.99 | 64.96 | < 0.001 |
90% (D90) | 78.64 | 119.02 | < 0.001 |
80% (D80) | 93.09 | 141.78 | < 0.001 |
50% (D50) | 134.79 | 206.17 | < 0.001 |
The HI with respect to 131Cs and 125I were 0.312 (range, 0.15-0.50) and 0.113 (range, 0-0.34), respectively (p < 0.001). The CI for 131Cs and 125I were 1.31 (range, 0.71-4.12) and 1.92 (range, 0.90-3.92), respectively (p < 0.001). These results are summarized in Table 3.
Discussion
The use of permanent brachytherapy seed implants for the treatment of brain metastases is advantageous, since it can provide a treatment to a highly localized area. This benefit allows the physician to precisely target a defined treatment volume and to avoid the surrounding tissues from potential damage. Permanent brachytherapy offers several more advantages compared to WBRT and SRS. The use of post-operative SRS may require a timeframe of 2-6 weeks after surgical resection in order to facilitate wound healing and stabilization of the cavity [4]. This delay in treatment may facilitate tumor cell repopulation. Similarly, concerns regarding the non-localized nature of WBRT and its toxicity profile have likely contributed to the modest decrease in WBRT use. For instance, WBRT’s acute toxicity profile includes fatigue, edema, nausea, vomiting, and decreased appetite [13]. While these acute effects are typically resolved spontaneously or can be effectively treated, the late toxic effects of WBRT are potentially more dangerous. This includes potentially irreversible neurocognitive damage, leukoencephalopathy, and radiation necrosis [12]. Permanent brachytherapy also offers a quality of life benefit that must be considered. Both the tumor resection and subsequent seed implantation occurs during one procedure, which is likely to increase patient satisfaction and convenience. These reasons, along with its cost benefits, provide justification for the increased interest in permanent brachytherapy. Given this context, we believe our results have a widespread applicability and may inform future clinical decisions.
There have been several studies reported in the literature employing the use of brachytherapy seeds for the treatment of brain metastases in the post-resection setting. Several studies have focused on the use of temporary 125I seeds, which allow for high-dose-rate delivery; however, many of these studies were performed after biopsy alone, and patients did not receive maximal possible resection. The more recent studies have used permanent brachytherapy seeds, of which the majority of them employed 125I. Unfortunately, most of these studies are retrospective in nature, and therefore encompass a diverse patient cohort and may be influenced by selection bias. Furthermore, these studies often include patients with both newly diagnosed brain metastases and those with recurrences. Taking these factors into account, the reported median survival for the treatment of newly diagnosed brain metastases ranges from 9.3-17.8 months and is associated with local control rates of 60-100%, with radiation necrosis rates of 0-26% [11]. These data compare favorably to surgical resection plus SRS in terms of both the median survival of 14 months as well as the local control rates of 85% [14].
The main criticism against the widespread adaptation of brachytherapy for the treatment of brain metastases is the high incidence of RN that has historically been associated with this technique [9]. Rogers et al. reported 23% rate of radiation necrosis when using high-dose temporary brachytherapy, such as the IsoRay Gliasite balloon [15]. Using continuous low-dose permanent 125I brachytherapy, Bogart et al. reported 0% rates of radiation necrosis, yet only achieved 80% rate of local control [16]. Alternatively, Huang et al. reported 26% rate of radiation necrosis, also using permanent 125I brachytherapy, while achieving 95% local control rate [12]. The disparity in results between these two series can be understood if attention is given to the activity and dose prescribed in each case. Bogart et al. employed seeds with activity of 0.32-0.45 mCi and a cumulative dose of 80-160 Gy [15], while Huang et al. implanted seeds with a median activity of 0.79 mCi and delivered a median dose of 400 Gy to a depth of 5 mm [12]. Based on these data, Huang et al. concluded that decreasing both the seed activity as well as the prescription dose will likely result in a decrease of RN, while minimally impacting local control rates. The prospective trial for 131Cs was carefully designed to take into account the aforementioned factors in an attempt to limit the incidence of RN while maintaining local control. The lowered seed activity of 131Cs and our utilized dose prescription not only achieved a high-rate of local control (100%), but also resulted in zero incidences of RN [8].
In addition to the study design that was used when employing 131Cs, the physical characteristic of creating more lower dose gradient isodose lines to the source has likely contributed to its success. In this analysis, we have shown that even when using the same prescription dose parameters as 131Cs, 125I allows for significantly higher amount of brain tissue being exposed to radiation. Apart from irradiating lower volumes of brain tissue, 131Cs also delivers significantly lower doses to equivalent volumes of CTV when compared to 125I. Additionally, the higher HI of 131Cs indicated more uniform dosing within the CTV. Lastly, the CI of 131Cs was closer to 1, indicating that the prescription dose was delivered more accurately to the desired volume of CTV. With 125I, not only was there less uniformity of dosing within the CTV, but the total volume receiving the prescription dose was also nearly double the desired volume of CTV. We believe that these factors may be particularly pertinent when looking at RN as an endpoint. Dagnew et al. stated that in their study, both patients who presented with symptomatic RN had lesions that exceeded 3 cm in diameter [11]. Due to the size of their lesions, a greater number of seeds were required to cover the cavity, resulting in a total radiation activity greater than 40 mCi. These results suggest a dose-dependent risk of RN. More recently, Petr et al. reported 6% incidence of RN while using permanent 125I brachytherapy seeds in the setting of post-resected brain metastases [17]. It was noted that the patients in the study had relatively large metastases, and specifically all the patients that developed RN had lesions larger than 3 cm. Therefore, when compared to 125I, 131Cs limits the surrounding brain tissue from additional radiation, restricts dose delivery within the CTV, provides more uniform dosing within CTV, and accurately targets the desired area. These factors, in turn, may contribute to minimizing the incidences of RN. These characteristic of 131Cs probable allow it to be utilized even when dealing with cavities > 3 cm.
Some studies have demonstrated an improved control with dose escalation [18,19,20], particularly in tumors receiving an equivalent dose in 2 Gy fractions (EQD2) > 35 Gy (α/β = 10) or biological effective dose using an α/β = 12 (BED 12) > 40 Gy (linear quadratic cubic model). There are newer prospective reports of using GammaTile (a 131Cs radioisotope embedded into collagen), which utilizes very high doses of radiation, similar to our studies. The doses of 80 Gy prescribed to 5 mm depth are extracted from the use of 131Cs in the prostate. Dose escalation in brain metastases and primary brain tumors appear promising in the radiation literature. We have utilized a conversion from dose from low-dose-rate to high-dose-rate, using a dose factor of 0.54 [21].
It must be noted that dosimetric superiority may not always translate into better clinical outcomes. Prospective studies, comparing the long-term clinical outcomes of 131Cs and 125I, need to be performed to elucidate this point. Despite this, our RN levels of 0% with 131Cs are very encouraging and may provide a glimpse into its potential long-term clinical benefits compared to 125I. It must also be noted that RN is a multifactorial complication and likely depends not only on the inherent dosimetric characteristics of the radioisotope, but also on study-specific factors, such as the prescribed dose levels, seed activity levels, etc. It should also be mentioned, that because the strength and position of the 125I sources were not optimized, it could contribute to the quality of its dosimetry.
Another feature that impacts the incidence of RN is the length of time, over which the seed can deliver its intended dose as well as the dynamics of the cavity during that time. It was recently reported that the cavity size is not significantly reduced in size during the 33-day period, during which 131Cs delivers 90% of its intended dose [22,23]. However, when dealing with an isotope, such as 125I, which takes 120 days to deliver the equivalent dose, a cavity may undergo significant shrinkage. When the cavity does undergo shrinkage, this means that the prescription dose is being delivered to a larger volume of surrounding tissue over time rather than the intended CTV volume. We believe that this fact may also contribute to the increased incidence of RN, when employing 125I compared to 131Cs.
Conclusions
In this study, we report that 131Cs permanent brachytherapy for the treatment of brain metastases exposes lower volumes of brain tissue to radiation, provides more uniform dosing within CTV, and targets the desired CTV volume more accurately, when compared to 125I. These findings coupled with advantageous physical characteristics of 131Cs, namely a shorter T1/2, shorter time of dose delivery, minimal volume of cavity shrinkage, and more compact dose distribution, provide a dosimetric superiority to 125I. The results of this analysis may provide a rationale for the 0% incidence of RN, when treated with maximally safe neurosurgical resection and intra-operative 131Cs implantation in patients with newly resected brain metastases.