Purpose
Current standard of care therapy for locally advanced cervical cancer includes a combination of external beam radiation and brachytherapy boost given concurrently with platinum-based chemotherapy [1-4]. Brachytherapy is a necessary component of curative intent therapy, and results in an increased survival compared with external beam boost techniques [5-7]. Historically, brachytherapy treatment planning has been performed using standard orthogonal X-ray images. Radiation dose was prescribed to points defined by a brachytherapy applicator without the use of volumetric computed tomography (CT) or magnetic resonance imaging (MRI) to define target volumes [8]. More recently, the Groupe Européen de Curiethérapie-European Society for Therapeutic Radiology and Oncology (GEC-ESTRO) provided recommendations for image-guided adaptive brachytherapy utilizing CT or MRI imaging [9, 10]. These techniques have been applied in a prospective clinical trial, EMBRACE (External beam radiochemotherapy and MRI-based adaptive BRAchytherapy in locally advanced CErvical cancer), with recently reported outcomes demonstrating primary tumor control rates of 92%, with cumulative incidence of grade 3-5 toxicities below 15% [10-12].
While utilization of the GEC-ESTRO planning concepts [13, 14] has led to improvements in the quality of patient care, additional questions remain. With the use of advanced imaging techniques and more labor-intensive treatment planning, typical delays of 2-4 hours may occur between acquisition of treatment planning images and treatment delivery [15, 16]. In addition, the location where MRI imaging is performed is often physically distant from the brachytherapy afterloader for high-dose-rate brachytherapy, requiring patient transport between acquisition of treatment planning scans and therapy. These issues create potential for applicator displacement and associated differences in planned versus administered therapy. At present, there are no agreed upon best practice standards for applicator immobilization, even though various techniques are used in clinical practice, including placement of gauze packing in the vagina, suturing the applicator into place, or using external fixation clamps. Likewise, there are no agreed upon best practices for pre-treatment imaging to verify applicator position immediately prior to treatment. Relatedly, there is little published data to guide radiation oncologists regarding clinical significance of an observed applicator displacement or tolerance limits that should be applied before applicator re-positioning and/or re-planning. Additional quantitative information is needed to guide clinicians during administration of brachytherapy treatments.
Previous single institution modeling studies utilizing intracavitary (IC) applicators have demonstrated that small displacements in applicator positioning may lead to substantial alterations in dosimetry [17, 18]. However, these studies included relatively small numbers of patients and the data has not been corroborated. In addition, recent trends in image-guided and adaptive brachytherapy for cervical cancer have emphasized increased utilization of hybrid or combined intracavitary and interstitial (IC/IS) applicators, which allow greater radiation dose escalation to high-risk clinical target volume (HR-CTV) and greater sparing of radiation dose to adjacent OARs (organs at risk). The impact of applicator displacement on IC/IS dosimetry has, to our knowledge, not been previously reported. Therefore, in the present paper, we sought to determine the impact of applicator displacement utilizing current best practice treatment planning concepts for image-guided and adaptive brachytherapy in a patient population treated with a combination of IC and IC/IS brachytherapy applicators.
Material and methods
Patient cohort
This was a review of 27 consecutive patients receiving IC or IC/IS brachytherapy boost during definitive treatment for cervical cancer at a single academic medical center between 2019 and 2021. The randomized patient cohort was based on brachytherapy treatments administered using either the Geneva or Venezia tandem and ovoid IC/IS brachytherapy applicator [19-21] (Elekta, Inc.). For each brachytherapy treatment, an IC or IC/IS tandem and ovoid applicator was placed under anesthesia by an experienced physician using standard methods [8, 22]. The type of anesthesia utilized (monitored anesthesia care or general anesthesia) was chosen by the managing anesthesiologist on the day of treatment, and CT images (Phillips BrillianceTM, Big Bore 16-slice CT scanner) with a 2 mm slice thickness were obtained, with a protocol of 120 kV and 500 mAs per slice and a 50/60 cm field of view. MRI images were acquired with a 2 mm T2 modality using Siemens SkyraTM 3T or General Electric HDXTTM 1.5 T scanners. Imaging field of view was approximately L5/S1 interspace through the perineum. Utilization of IC vs. IC/IS applicators was according to a physician discretion based on primary tumor characteristics and patient anatomy at the time of brachytherapy applicator placement. CT/MRI images were then transferred to Oncentra 10.0 (Elekta, Inc.) treatment planning system (TPS). According to the GEC-ESTRO recommendations, a radiation oncologist specializing in gynecologic brachytherapy contoured the HR-CTV and OARs, including the bladder, rectum, sigmoid colon, and any loops of the small bowel within 2 cm of the HR-CTV. Contoured CT/MRI images were used to create 3-dimensional brachytherapy (3D-BT) treatment plans for each patient. Choice of treatment planning imaging (CT or MRI) was per physician discretion. During radiation therapy for cervical cancer, the total physical radiation doses for 3D-BT and external beam radiation are summed and normalized to an equivalent dose at 2 Gy per fraction (α/β = 10 Gy for tumor, and α/β = 3 Gy for organs at risk) [10]. In order to be representative of a single fraction from a 30 Gy in 5 fraction brachytherapy boost regimen for cervical cancer, treatment plans were optimized to achieve a dose of 6 Gy to the HR-CTV D90% while minimizing radiation dose to D2cc for adjacent OARs. All the dose calculations were done on Oncentra Brachy treatment planning system based on the American Association of Physicists in Medicine (AAPM) TG-43 protocol [23, 24]. Descriptive statistical results for the HR-CTV and OARs for the 27-patient cohort are shown in Table 1, which presents the mean (μ) ± standard deviation (σ) of the planned dose for D90%, and the received doses for D0.1cc, D1cc, and D2cc (as a percentage of the HR-CTV D90% prescribed dose) for OARs across the 27 patients.
Table 1
Dosimetric parameters with means and standard deviations for the high-risk clinical target volume (HR-CTV), rectum, and bladder across all 27 patients. Doses to organs at risk (OARs) are presented as a percentage of prescribed dose to the HR-CTV D90%
To analyze the impact of applicator displacement on the dose to the HR-CTV and OARs, brachytherapy applicators in clinically optimized plans were virtually shifted in the inferior direction by 2 mm, 5 mm, 7 mm, and 10 mm. The minimal shift of 2 mm was based on previously published literature on toxicity analysis, which showed that 2 mm longitudinal shift in applicator can have a 5.6% relative standard deviation for rectum D2cc, where some other studies estimated 1.625-3.25 mm shifts for a dose uncertainty in 1-2% [25-27]. Therefore, shift increments of 2-3 mm from clinically optimized plan up to 10 mm were utilized in the current investigation. The inferior direction was chosen as the most likely direction of displacement, given that the applicator and ovoids were bounded by the upper vagina mucosa laterally, and the tandem was fixed within the cervical canal, preventing lateral displacement. In addition, appropriate positioning of the ovoids abutting the cervix/vaginal fornices physically prevented superior displacement of the applicator. For each virtual shift, all brachytherapy source dwell position, including interstitial needle dwell positions in combined IC/IS cases, were shifted uniformly without adjusting dwell times. Out of the total patients analyzed, 63% (n = 17) received IC brachytherapy, whereas 37% (n = 10) received IC/IS brachytherapy. A separate analysis was performed comparing HR-CTV D90% doses for IC vs. IC/IS applicators. Separate statistical analyses were done, and percentage deviations and p-values were calculated for each scenario. Treatment planning was performed using iridium-192 with source position step sizes of 5 mm [28]. In one patient, the virtual applicator shifts resulted in the modeled applicator ovoid positions overlapping directly with an OAR. In this case, the involved OAR was re-contoured, with cropping portions of the OAR overlapping with the modeled ovoids, to account for deformation that would occur by the applicator. A representative image demonstrating a virtual applicator displacement and associated shifts in radiation dose distribution is shown in Figure 1. For each modeled applicator displacement, dose volume histogram (DVH) analyses were performed to determine effects on D90% of the HR-CTV and D2cc of the bladder, rectum, and sigmoid.
Fig. 1
A) Sagittal view of clinically optimized radiation plan showing high-risk clinical target volume (HR-CTV; red-dashed) and surrounding OARs (rectum: brown-dashed, bladder: yellow-dashed, sigmoid: pink-dashed, and bowel: green-dashed). The tip of applicator is shown in horizontal line demarcated as ‘Clinical Position’. B) Virtually simulated plan showing the tip of applicator shifted from clinical position 10 mm in the inferior direction. Distances between source positions are 5 mm

Statistical analysis
A comprehensive statistical analysis was performed using Statistical Analysis SystemTM (SAS v. 9.4) software suite. Levene’s test [29] was applied, and if it indicated that the equal variances were not true, non-parametric Kruskal-Wallis (KW) test was carried out to determine the clinical significance of each displacement [30, 31], organ, and volume levels for OARs. Otherwise, to calculate the mean values and standard deviations of the mean for each displacement, organ, and volume levels for OARs, a general linear model (GLM) was used. Two different types p-values were estimated, such as one type demonstrated overall significance from KW test or GLM type III F-test, and the other type showed pair-wise multiple comparison significance. Under KW test, pair-wise two-sided multiple comparison analysis using DSCF (Dwass, Steel, Critchlow-Fligner) method was performed, and under GLM, Bonferroni adjustment for multiple comparison was applied.
Results
Dosimetric impact of applicator displacement on hr-ctv
As demonstrated in Table 2, the mean doses of HR-CTV D90% decreased by 4.2%, 11.1%, 15.4%, and 22.9% at modeled displacements of 2, 5, 7, and 10 mm, with respect to the D90% of clinically optimized plan. Displacements of 5 mm or greater were statistically significant. A DVH for the HR-CTV at clinical position and modeled shifts up to 1 cm is shown in Figure 2. The mean D90% dose, standard error, and 95% confidence limits are shown in Figure 3A. To provide estimates for displacements values not specifically evaluated in our study, data were fitted with first order linear regression and found to have a Pearson correlation coefficient of R2 ~0.99 (Figure 3B).
Fig. 2
High-risk clinical target volume (HR-CTV) DVH analysis for different virtual shifts. The prescription for the patient was 6 Gy prescribed to D90% of HR-CTV, as demonstrated by the point of intercept with vertical dashed line in in clinical position. The proportion of HR-CTV receiving 6 Gy was reduced to 87%, 82%, 78%, and 72% at modeled shifts of 2, 5, 7, and 10 mm, respectively
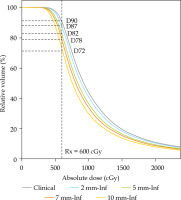
Fig. 3
Box-whisker plot for mean high-risk clinical target volume (HR-CTV) D90% dose as a percentage of prescribed dose for each virtual shift with respect to clinical position (A). Boxes represent standard error, with the midline of the box showing median distribution, and whiskers representing 95% confidence intervals for clinical, 2 mm, 5 mm, 7 mm, and 10 mm shifts. This clearly shows the under coverage of HR-CTV for inferior applicator displacements. B) The mean D90% and associated standard error fitted with a first order linear polynomial. The horizontal dashed line shows the threshold displacement (2 mm) where beyond significant under coverage can occur for HR-CTV
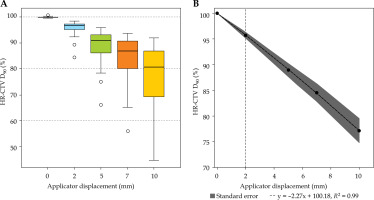
Table 2
Mean and standard deviations of D90 dose to the high-risk clinical target volume (HR-CTV) for individual shifts. Corresponding p-values were calculated pair-wise with respect to unshifted position. D90% differences are calculated with respect to clinical plan as 100 × (DShift 90 – DClinical 90) divided by DClinical 90, where shift = 2, 5, 7, 10 mm. P-value < 0.05 indicate a clinically significant change
Displacement | Dose (%) | p-value | D90% difference | |
---|---|---|---|---|
Mean | SD | |||
Clinical | 99.96 | 0.27 | ||
2 mm | 95.73 | 2.99 | 0.4240 | 4.2 |
5 mm | 88.90 | 6.76 | < 0.0001 | 11.1 |
7 mm | 84.56 | 9.10 | < 0.0001 | 15.4 |
10 mm | 77.11 | 12.24 | < 0.0001 | 22.9 |
Dosimetric impact of applicator displacement on oars
Dose volume parameters (D0.1cc, D1cc, and D2cc) were analyzed for the rectum, sigmoid, and bladder for each modeled displacement, and compared with the clinical position. Table 3 shows the mean and standard deviations for doses to the rectum in clinical position and for modeled virtual shifts. Doses were written as a percentage of the dose prescribed to the HR-CTV D90%. For the rectum, virtual displacements of greater than 5 mm resulted in statistically significant increases in D2cc values, while shifts of 5 mm or greater were significant for D1cc and D0.1cc (Table 3). A box-whisker plot for each rectal dose/volume parameter is depicted in Figure 4, where a clear increase in D90% is observed for all virtually simulated shifts. Specifically, the mean rectal D2cc dose increased by 6.75% for the 2 mm shift, and 44.10% for the 10 mm shift. Relative to D2cc and D1cc, D0.1cc doses were more sensitive to applicator shifts (70% for the 10 mm shift). We found that that the rectum was the OAR most affected by applicator displacements, as we found no increases in dose to the bladder or sigmoid with modeled applicator displacements. In fact, displacements led to clinically favorable changes in radiation doses to the bladder and sigmoid, with a trend towards decreasing D2cc, D1cc, and D0.1cc doses with greater applicator displacements, as shown in Figure 5. This was due to the applicator dwell positions moving inferiorly away from the bladder and sigmoid, with inferior applicator displacements as modeled in the current study.
Fig. 4
Box-whisker plots for rectum D0.1cc, D1cc, and D2cc doses for each virtual shift with respect to clinical position. Doses are presented as a percentage of prescribed dose to high-risk clinical target volume (HR-CTV) D90%. Boxes represent standard error, with the midline of the box showing median distribution, and whiskers representing 95% confidence intervals for clinical, 2 mm, 5 mm, 7 mm, and 10 mm shifts. Outlier values are depicted as circles

Fig. 5
Box-whisker plots for the bladder (A-C) and sigmoid (D-F) D0.1cc, D1cc, and D2cc for each virtual shift with respect to clinical position. Doses are presented as a percentage of prescribed dose to high-risk clinical target volume (HR-CTV) D90%. Boxes represent standard error, with the midline of the box showing median distribution, and whiskers representing 95% confidence intervals for clinical, 2 mm, 5 mm, 7 mm, and 10 mm shifts. Outlier values are depicted as circles
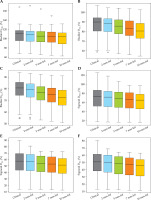
Table 3
Mean and standard deviations for the rectal D0.1cc, D1cc, and D2cc for individual shifts. Corresponding p-values were calculated pair-wise with respect to unshifted position. Values are provided as a percentage of prescribed dose to the high-risk clinical target volume (HR-CTV) D90%
Intracavitary vs. interstitial cases
Combined IC/IS brachytherapy applicators allow more conformal radiation doses distributions and greater sparing of adjacent OARs compared with IC applicators, leading to a hypothesis that IC/IS applicator displacements may result in relatively greater alterations in radiation doses to target volumes and OARs compared with IC applicators. To test this hypothesis, we compared the HR-CTV D90% for IC vs. IC/IS applicators. Similar to the overall patient cohort, any displacements of 5 mm or greater resulted in significant decreases in dose to the HR-CTV D90% for both IC and IC/IS applicators. At all modeled displacements, numerically greater reductions in dose to the HR-CTV D90% were observed with IC/IS as opposed to IC applicators (Table 4).
Table 4
Mean and standard deviations of D90% dose to the high-risk clinical target volume (HR-CTV) for individual shifts for IC vs. IC/IS brachytherapy applicators. Corresponding p-values were calculated pair-wise with respect to unshifted position
The mean values and standard errors for the HR-CTV D90% dose and rectal D2cc fitted with first degree polynomials are shown in Figure 6. A clear difference can be observed for a dose to the HR-CTV D90%, with IC/IS cases showing greater reductions relative to IC, as presented in Figure 6A. For both IC/IS and IC cases, significant differences in rectal D2cc doses occurred with displacements beyond 7 mm. As with the combined IC and IC/IS patient population, we observed no increases in doses to the bladder and sigmoid when IC and IC/IS displacements were evaluated individually. As shown in Figure 6B, the mean D2cc was numerically greater for IC/IC cases, but the standard error was intercepting, which demonstrated that the differences were not statistically significant.
Fig. 6
Mean high-risk clinical target volume (HR-CTV) D90% and associated standard error fitted with a first order linear polynomial for IC and IC/IS cases (A). The horizontal dashed line shows the threshold displacement (2 mm) where beyond which statistically significant under coverage occurs. The mean rectum D2cc with standard error is fitted with second order polynomials (B). The horizontal dashed line shows the threshold displacement of 7 mm beyond which statistically significant differences were observed
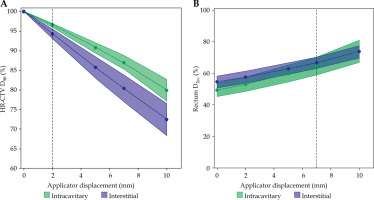
Discussion
There is little published data assessing the dosimetric impact of brachytherapy applicator displacements during image-guided adaptive brachytherapy for cervical cancer [17, 18, 32]. Our findings demonstrate the clinical significance of even small shifts in IC or IC/IS applicator position, and closely reflect previous studies evaluating IC applicators alone. Schindel et al. published their analysis of 20 patients treated with IC brachytherapy using a tandem and ovoid applicator [18]. Similar to our data, they found significant differences in doses to OARs with applicator displacements beyond a few mm in the inferior direction. Of note, they observed an approximate 50% increase in the dose to the rectal D2cc with displacements of 10 mm, which very closely matches our data, showing a 44% increase in rectal D2cc at 10 mm displacement. However, our findings differ substantially with respect to changes in the dose to the HR-CTV. Schindel and colleagues found a non-significant 4% decrease in the dose to the HR-CTV D90% with modeled displacement of 10 mm [18]. In contrast, we found statistically significant differences at displacements of 5 mm or greater, with an approximate 23% difference in the dose to the HR-CTV D90% at 10 mm of displacement. A similar study performed by Wu et al. modeled displacements for tandem and ovoid applicators, and found reductions in the dose to the HR-CTV D90% of approximately 15% at 10 mm inferior displacement [17]. The cause for this discrepancy in results between the two studies is not clear, though possibilities would include institutional differences in planning practices, inter-provider differences in contouring technique, and difference in brachytherapy applicator geometry. For example, modeled displacements using of a tandem with a 15 degree vs. 30 degree angle could results in significantly different shifts in applicator reconstruction with respect to CTV. This also suggests a potential limitation of the current study in that the HR-CTV volume does not deform with modeled tandem displacement as may occur with physical displacement within a patient.
To the best our knowledge, this study represents the first assessment of the dosimetric impact of brachytherapy applicator displacement for IC vs. IC/IS applicators. Data from retro-EMBRACE study have demonstrated that the use of IC/IS vs. IC applicators is associated with greater conformality, increased dose to the HR-CTV D90% with improved local control, and no differences in OAR D2cc doses or rates of late toxicity [33]. IC/IS applicators have been increasingly utilized in clinical practice, particularly for large or irregular HR-CTV volumes [33]. However, our findings suggest that IC/IS applicators may be particularly prone to under-coverage of the HR-CTV in comparison with IC applicators. This may in part explain the relatively greater differences in HR-CTV dose reduction observed in the present study relative to those of Schindel et al. and Wu et al. Another potential limitation of our work is that we were unable to account for the effect of displacement for different HR-CTV volumes. It is possible that larger or smaller HR-CTV volumes would be more affected; however, our study lacks sufficient sample size to fully evaluate this possibility, which would be an interesting area for future research.
The findings of the present study demonstrate the importance of careful monitoring for shifts in applicator position between the acquisition of treatment planning imaging and the treatment delivery; however, there are relatively few studies assessing the degree of applicator displacement that occurs in clinical practice. Most efforts to characterize applicator displacement between the time of treatment planning imaging and the treatment delivery have utilized orthogonal X-rays and measuring shifts in position of the applicator in relation to bony landmarks, with observed displacements usually less than 1 cm [34-36]. Our findings show that shifts within this range may lead to clinically meaningful changes in dosimetry. However, these studies are limited, since they do not provide an assessment of changes in applicator position relative to the cervix, rectum, bladder, or sigmoid directly, which would require 3-dimensional imaging with CT or MRI, rather than orthogonal X-rays. Providers may utilize various techniques to minimize applicator motion and reduce displacement errors, including vaginal packing, external fixation clamps, and pre-treatment imaging. There is little data to demonstrate superiority of any technique over another, or to establish a clear best practice. In addition, there is little published data confirming the directions, in which displacements are most likely to occur (anterior/posterior, left/right, superior/inferior, or rotational). Here, we chose to focus on inferior displacements as we felt this was the most representative of what may occur anatomically, with displacement of the applicator away from the cervix along the vaginal canal. Displacements in the lateral direction would be prevented by the ovoid abutment of the vaginal wall as well as the tandem abutment of the endocervix, and superior displacements would be prevented by the ovoids abutting the vaginal fornices.
Conclusions
A comprehensive systematic analysis was performed to understand the dosimetric influence of applicator displacements along the inferior directions in 3D cervical cancer brachytherapy. The results indicated that the HR-CTV D90% is highly sensitive, with more than 2 mm displacements resulting in clinically significant underdosage, particularly for IC/IS applicators. Additionally, the rectum was the most impacted OAR, with any displacement beyond 5 mm leading to significantly higher dose. Specifically, we found that rectum D0.1cc is the most sensitive to applicator displacements, compared with D1cc and D2cc. Our results demonstrate the importance of applicator immobilization techniques and careful monitoring of applicator position during planning and administration of image-guided adaptive brachytherapy for cervical cancer.