Introduction
Aseptic necrosis of tissues is a common and often neglected injury that occurs both in everyday life (sunburn, bruises) and in the clinic (postoperative). However, it has been shown that even seemingly innocuous injuries can impair host defense mechanisms [1]. We previously observed in an animal model that turpentine-induced tissue necrosis enhanced the febrile response to bacterial lipopolysaccharide (LPS) when injected after 48 hours [2]. This was associated with increased blood concentrations of fever mediators, such as interleukin 6 (IL-6) and prostaglandin E2 (PGE2), as well as high mobility group box protein 1 (HMGB1). Moreover, the blockade of HMGB1 activity with antibodies reduced the described hyperpyretic increase in body temperature. We therefore concluded that HMGB1 is a plasma-derived factor that is released in the course of aseptic injury and could enhance the pyrogenic effects of LPS.
HMGB1, which was discovered in the early 1970s [3], is a highly conserved protein present in the nucleus of almost all cell types. It plays an important role in maintaining the nucleosome structure and regulation of gene transcription [4, 5]. However, recent evidence has shown that HMGB1 mediates the immune response by functioning as a damage-associated molecular pattern (DAMP) molecule [6]. The release of HMGB1 into the extracellular matrix occurs through two main pathways: actively by inflammatory cells (i.e., endotoxin-stimulated monocytes and macrophages) and passively during cell necrosis and apoptosis [7]. The receptor for advanced glycation end products (RAGE) was the first receptor to bind HMGB1 [8]. In addition to RAGE, the Toll-like receptor family (TLR), found on the surface of immune and endothelial cells, has been shown to play an important role in HMGB1 signaling. The binding of HMGB1 to TLR2 and TLR4 leads to upregulation of nuclear factor κB (NF-κB), so stimulation of these receptors by HMGB1 can likely lead to cytokine release [9].
Here, we sought to determine whether injection of recombinant HMBG1 protein alone would sensitize rats to LPS, as did turpentine-induced aseptic necrosis in our previous study [2]. We also tested whether HMGB1 is involved in priming immune cells for further contact with pathogens. Therefore, we measured the release of fever mediators (IL-1β, IL-6, and PGE2) from LPS-stimulated peripheral blood mononuclear cells (PBMCs) isolated from rats injected with turpentine, HMGB1, or heat-denatured HMGB1. Nuclear factor κB activation in these cells was also determined.
Material and methods
Animals
Male rats (strain: Wistar, Crl: WI [Han]) aged 8-12 weeks and weighing 250 g to 300 g were purchased from the Mossakowski Medical Research Centre of the Polish Academy of Sciences (Warsaw, Poland) and acclimatized for 10 days after shipment before the beginning of experiments. Animals were kept individually in a room with constant relative humidity (60 ±5%), temperature (24 ±1°C), and a 12 : 12-hour light-dark photoperiod. Rodent laboratory food and drinking water were provided ad libitum. All procedures were approved by the local Animal Welfare Bioethics Committee (Approval No. 20/2016). Experimental procedures are depicted in Figure 1.
Injections
Turpentine oil (Elissa, Warsaw, Poland) was injected subcutaneously (s.c.) into the right hindlimb at a 0.1 ml/rat volume. LPS from Escherichia coli 0111:B4 (Sigma-Aldrich, St. Louis, MO, U.S.A.) was dissolved in pyrogen-free 0.9% sodium chloride (saline) and injected intraperitoneally (i.p.) at a dose of 50 µg/kg. Lipopolysaccharide is an exogenous pyrogen that when injected into animals provokes a characteristic and repeatable febrile response. Subcutaneous or intramuscular injection of a small amount of turpentine is a widely used model of aseptic and local inflammation that induces a robust acute phase response (APR) consisting of fever and acute phase protein production [10]. Furthermore, a gradual increase in plasma HMGB1 after injection of turpentine is observed [2]. Recombinant rat HMGB1 protein was reconstituted at a concentration of 100 µg/ml in sterile PBS and injected into the rat at a dose of 5 µg/rat. Heat-denatured (56°C for 1 h) HMGB1 protein was used as a control.
ELISA assays
Blood was collected by cardiac puncture into ethylenediaminetetraacetic acid solution (EDTA, Sigma-Aldrich, St. Louis, MO, USA) from anesthetized rats (ketamine/xylazine mixture). Plasma was separated by centrifugation (20 minutes, 1000 × g) within 30 minutes after collection. All samples were frozen at –20°C until assayed. Indomethacin (Sigma-Aldrich, St. Louis, MO, USA) in a final concentration of approximately 10 µg/ml was added to the blood samples before centrifugation and separation of plasma for measurement of PGE2 content. The levels of IL-6 and PGE2 content in plasma and cell supernatants were determined using ELISA kits from R&D Systems (Minneapolis, MN, USA). HMGB1 concentration in plasma was determined using an EIAab ELISA kit (Wuhan, China). NF-kB p65 activation in nuclear extracts was determined using the assay kit from Abcam (Cambridge, UK). The optical density was measured using a multidetection microplate reader (Synergy model HT; BioTek, Winooski, VT, USA).
Cell stimulation and nuclear extract isolation
Blood was collected by the same method as described above. Peripheral blood mononuclear cells were isolated by density gradient centrifugation; for details see ref. [11]. All procedures resulted in greater than 95% viable PBMCs in suspension by trypan blue dye (MP Biomedicals, Santa Ana, CA, USA) exclusion assay. Freshly isolated PBMCs were immediately seeded at an exact density of 1 × 106 cells/ml in 12-well cell culture plates. Cells were preincubated for 1 hour in sterile RPMI medium 1640 (Cytogen GmbH, Sinn, Germany) containing 2 mM L-glutamine supplemented with heat-inactivated 10% fetal bovine serum (FBS, PAN Biotech, Aidenbach, Germany) in the presence of a solution of streptomycin and penicillin (Biochrom, Berlin, Germany) at 37°C, 5% CO2, and 95% humidity. After preincubation, cells were stimulated for 4 hours with LPS (1 µg/ml/106 cells) diluted in a pyrogen-free saline solution. As a control, cells were incubated with 0.9% NaCl. After stimulation, cell suspensions were collected and transferred to sterile 2-milliliter microcentrifuge tubes and centrifuged at 1500 × g for 1 minute. The resulting supernatants were stored at -20°C until assayed by ELISA for the presence of IL-6, IL-1β, and PGE2. Nuclear extract of cells was obtained using the nuclear extraction kit (Abcam, London, UK; cat. no. ab113474) according to the manufacturer’s instructions. Total protein concentration in the samples was determined using the Pierce BCA Protein Assay Kit (Thermo Fisher Scientific, Waltham, MA, USA). The extent of NF-κB p65 activation was expressed as the ratio of measured absorbance (OD 450 nm) expressing quantified NF-κB p65 activation per 1 mg total nuclear protein.
Statistics
The mean ± standard error mean (SEM) of the concentrations of IL-6, IL-1β, PGE2, and HMGB1 in plasma was calculated for four plasma samples, each obtained from different animals in the experimental group and examined in duplicate. Cell supernatants assayed in duplicate for the presence of IL-6, IL-1β, PGE2, and HMGB1 were from three separate replicates of the same experimental variant. ANOVA with repeated measures followed by a Tukey multiple comparison post hoc test was used to detect statistical differences between groups. Differences were considered significant at p < 0.05. GraphPad Prism 10.0 software (GraphPad Software Inc., San Diego, CA, USA) was used to perform statistical comparisons between different values.
Results
Injection of HMGB1 protein provoked an increase in PGE2 level in the plasma of rats and augmented release of IL-1β after LPS administration
As can be seen in Figure 2A, pre-injection of recombinant HMGB1 protein caused an increase in IL-1β concentration observed 4 hours after LPS administration (576 ±12 pg/ml) that was almost 3-fold higher than in animals injected with heat-denatured HMGB1 before LPS (179 ±57 pg/ml) but not as high as in animals injected with turpentine 48 hours before LPS (906 ±172 pg/ml). All the injections in experimental groups are depiced in Figure 1A. The highest concentration of IL-6 was observed in the rats from the experimental group TRP/LPS (8898 ±263 pg/ml) was twice as high as in the HMGB1/LPS and HMGB1den/LPS groups (4387 ±414 pg/ml and 3418 ±449 pg/ml, respectively), as shown in Figure 2B. Rats injected with HMGB1 and NaCl produced negligible amounts of both cytokines. Moreover, the highest PGE2 concentration was noted in rats injected with HMGB1 and after 48 hours with LPS (1372 ±257 pg/ml) and was not significantly different (p > 0.05) from rats injected with turpentine before LPS (1172 ±132 pg/ml) but also from rats injected with HMGB1 and two days later with saline (1162 ±160 pg/ml) (Fig. 2C). The PGE2 concentration in animals injected with denatured HMGB1 and LPS (479 ±12 pg/ml) was significantly (p < 0.001) lower than in the other experimental groups.
Fig. 2
Changes in plasma levels (pg/ml) of IL-1β (A), IL-6 (B) and PGE2 (C) in rats pre-treated with turpentine, HMGB1 or heat-denatured HMGB1 and injected with LPS after 48 h. One group of rats was injected with HMGB1 and after 48 h with saline. Blood for ELISAs were collected 4 h after LPS injection. Values are means ±SEM. Letter n indicates sample size in respective group. Asterisk indicates significant difference (*p < 0.05, ***p < 0.001, respectively)

PBMCs isolated from rats injected with HMGB1 release to culture media increased amounts of IL-1β and PGE2 when stimulated with LPS
The experimental procedures are depiced in Figure 1B. Peripheral blood mononuclear cells isolated from animals injected with turpentine released the highest amount of IL-1β (578 ±38 pg/ml) when stimulated with LPS, twice as much as cells isolated from animals injected with HMGB1 (230 ±7 pg/ml), as shown in Figure 3A. Interleukin 1β release from cells isolated from rats injected with denatured HMGB1 (69 ±23 pg/ml) was comparable with the values measured for the cells isolated from rats injected with NaCl (51 ±1 pg/ml) (p > 0.05). Cells isolated from animals injected with turpentine released significantly higher amounts of IL-6 (1521 ±35 pg/ml) when stimulated with LPS than in the other experimental groups (corresponding to 292 ±4 pg/ml in the HMGB1; 251 ±5 pg/ml in the HMGB1den; and 281 ±7 pg/ml in the NaCl group), as shown in Figure 3B.
Fig. 3
Synthesis of IL-1β, IL-6 and PGE2 in the LPS- (A-C) or saline- (D-F) stimulated peripheral blood mononuclear cells (PBMCs) isolated from rats injected with turpentine, HMGB1, heat-denatured HMGB1 or saline. PBMCs were pre-incubated for one hour and then were stimulated with LPS (1 μg/ml/106 cells) or incubated with pyrogen-free saline for the next 4 h. Values are means ±SEM of IL-1β, IL-6 and PGE2 concentrations in the final culture medium from three independent experiments. Asterisk indicates significant difference (*p < 0.05, **p < 0.01, ***p < 0.001, respectively)
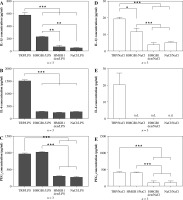
The highest amount of PGE2 was released from cells isolated from rats injected with HMGB1 (1023 ±26 pg/ml), similar to animals injected with turpentine (970 ±22 pg/ml) (p > 0.05). PGE2 concentration was comparable in the HMGB1den group (300 ±13 pg/ml) and in the NaCl group (269 ±26 pg/ml), as shown in Figure 3C. The release of IL-1β and IL-6 from cells incubated with NaCl was not significantly increased in all experimental groups (Fig. 3D and E). We also found that only cells isolated from rats injected with turpentine and cultured with NaCl released measurable amounts of IL-6 (20 ±7 pg/ml), as shown in Figure 3E. On the other hand, cells from these animals released the highest amounts of IL-1β (19 ±1 pg/ml), almost twice as much as cells from animals injected with HMGB1 (11 ±2 pg/ml) (p < 0.05). The amount of IL-1β was significantly (p < 0.01) lower in the case of cells isolated from rats treated with denatured HMGB1 (4 ±1 pg/ml) as well as with NaCl (5 ±0.4 pg/ml), as shown in Figure 2D. Such differences were also observed in the case of PGE2 (see Fig. 3F). Cells isolated from rats injected with turpentine and HMGB1 cultured with NaCl released similar amounts of PGE2 (corresponding to 413 ±12 pg/ml and 404 ±17 pg/ml; p > 0.05) but significantly (p < 0.001) more than cells isolated from rats injected with denatured HMGB1 (96 ±52 pg/ml) and NaCl (105 ±48 pg/ml).
NF-κB p65 activation in nuclear extracts
As shown in Figure 4, the highest activation of NF-κB was measured in nuclear extracts of cells isolated from animals injected with turpentine and stimulated with LPS (0.060 ±0.003). Slightly, but statistically significantly (p < 0.05), lower values were measured for the cells isolated from rats injected with HMGB1 (0.050 ±0.001) and the animals treated with HMGB1den (0.046 ±0.001) or NaCl before LPS stimulation (0.043 ±0.002). Activation of NF-κB was comparable in nuclear extracts of cells isolated from rats injected with turpentine (0.042 ±0.002) and HMGB1 (0.039 ±0.001) and incubated with NaCl. However, it was significantly (p < 0.001) higher in both groups in comparison to HMGB1den/NaCl (0.026 ±0.001) and NaCl/NaCl (0.028 ±0.001) experimental groups. Pairwise comparison between cells stimulated with LPS and incubated with NaCl revealed that the activation of NF-κB was significantly (p < 0.001) higher in LPS-stimulated cells isolated from rats injected with TRP or HMGB1 and cells incubated with NaCl from all experimental groups. In contrast, NF-κB activation was significantly (p < 0.001) lower in cells isolated from rats injected with denatured HMGB1 or saline and incubated with NaCl in comparison to all other LPS-stimulated cells. NF-κB activation in cells isolated from rats injected with turpentine or HMGB1 and incubated with NaCl was comparable to LPS-stimulated cells isolated from rats injected with NaCl or denatured HMGB1.
Fig. 4
Level of NF-κB p65 activation measured in PBMCs isolated from rats belonging to the same experimental groups as described in Fig. 3. Values are mean ±SEM of NF-κB p65 activation in the nuclear extracts obtained after cell stimulation per 1 mg of total nuclear protein. Asterisks denote significant differences between cells isolated from experimental groups and stimulated with LPS or incubated with saline separately (*p < 0.05, **p < 0.01, ***p < 0.001, respectively) whereas hashes represent significant differences between those groups (#p < 0.05, ###p < 0.001, respectively)
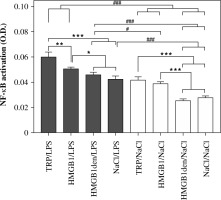
Discussion
Recent findings support the thesis that HMGB1 mediates the immune response to infection and inflammation by acting as a molecule of the damage-associated molecular pattern (DAMP) that is passively released from necrotic cells. In addition, HMGB1 can also be actively secreted by stimulated macrophages or monocytes [6, 12]. HMGB1 can increase the release of pyrogenic cytokines from cells by activating TLRs [13] and stimulating the synthesis/release of prostaglandins [14, 15]. Therefore, this protein can be considered to be involved, at least in part, in the mechanisms of monocyte/macrophage activation and priming. Whereas macrophages were shown to be responsible for the early stages of fever [16], we found that LPS-triggered fever was markedly increased 48 hours after injection of turpentine, and the early phase was especially enhanced and accelerated. This particular hyperpyretic effect was accompanied by a significant increase in plasma concentrations of fever mediators (PGE2 and IL-6), as well as HMGB1 protein (measured 4 hours after LPS administration). Furthermore, injection of HMGB1 antibody reduced hyperpyrexia, clearly showing that HMGB1 may be considered a crucial factor involved in the mechanism of sensitization to the pyrogenic effects of LPS [2].
Here, we investigated whether recombinant HMGB1 injected alone sensitizes rats to further contact with LPS. To this end, we measured blood concentrations of IL-1β, IL-6, and PGE2 4 hours after administration of LPS to rats pre-treated with turpentine or recombinant HMGB1 (or heat-denatured recombinant HMGB1 as a control). The concentration of these mediators was also measured in rats pre-injected with HMGB1 and then with saline (as a control for LPS). We found no statistically significant differences in fever mediator concentrations between animals from the HMGB1den/LPS group and animals pre-injected with saline and after 48 hours injected with LPS (data not shown).
We found that injection of recombinant HMGB1 in rats treated with LPS caused an increase in plasma concentrations of IL-1β and PGE2 but not of IL-6 (Fig. 2A and B). We also found a significant increase in plasma PGE2 levels after injection of HMGB1 alone (Fig. 2C) compared with heat-denatured HMGB1. This is consistent with previous reports showing that extracellular HMGB1 strongly enhances the cytokine response elicited by other pro-inflammatory molecules such as lipopolysaccharide and that HMGB1 alone could not induce detectable IL-6 production [17]. The authors emphasized that complex formation (HMGB1-LPS) is critical to achieving such an effect and that cytokine production can be inhibited by specific receptor blockade, suggesting that the synergistic effects are mediated by the partner ligand-reciprocal receptors TLR4 and IL-1RI, respectively [17, 18].
In our previous experiments, the fever response in rats previously injected with turpentine (associated with the release of HMGB1 into the bloodstream) was not only higher but also started almost immediately after LPS administration [2]. Therefore, we tested the hypothesis that HMGB1 is involved in priming PBMCs for enhanced activation in response to LPS. We found that PBMCs isolated from animals injected with turpentine but also with HMGB1 released increased levels of IL-1β and PGE2 but not IL-6 (Fig. 3A-C) into the culture medium when stimulated with LPS in vitro. We also noted greater activation of NF-κB in all cells stimulated with LPS compared with cells treated with saline. However, NF-κB activation was significantly higher in the saline-treated cells derived from animals injected with turpentine or HMGB1 compared with the other groups (Fig. 4B). This fact may explain why cells obtained from HMGB1-treated rats spontaneously produced increased IL-1β levels (Fig. 3D).
This is consistent with previous reports showing that HMGB1 promotes the synthesis of pro-IL-1β and pro-IL-18 in THP-1 macrophages by activating the MAPK pathway and NF-κB via RAGE receptors. He and co-workers [19] hypothesize that HMGB1 most likely plays an important role in the first step of IL-1β and IL-18 release, priming the excessive release of IL-1β and IL-18 by other cytokines that promote inflammation. Others have shown that IL-1β and HMGB1 additively promote the release of inflammatory cytokines in human intervertebral disc (IVD) cells. It was also proved that TLR, RAGE, and NF-κB signaling was additively promoted by IL-1β and HMGB1 [20]. Moreover, stimulation of fibroblasts with HMGB1 in complex with suboptimal levels of IL-1β significantly increased cyclooxygenase (COX) 2 expression and PGE2 production compared with treatment with HMGB1 or IL-1β alone [15].
HMGB1 actions have been studied in many cell types with most studies centering on myeloid cells. It is known that lymphocytes are capable of synthesis of endogenous pyrogens (cytokines) and PGE2. However, because of the fact that mice without functional lymphocytes B and T still develop normal febrile responses to LPS and turpentine [21] the hyperpyretic response to LPS observed after aseptic necrosis of tissues seems also to be dependent on myeloid cells. It is known that host monocytes/macrophages can be reprogrammed into an opposing primed or tolerant state depending on the type and amount of external stimulants [22, 23]. Our results suggest that HMGB1, as a primary “danger signal”, can reprogram a host’s innate immune system and make it sensitive to secondary signals – pathogens.
Summary
Pure recombinant HMGB1 has been shown to have negligible or low pro-inflammatory activities that act as a “danger signal” and promote tissue repair. However, it forms highly inflammatory complexes, especially with LPS or IL-1β, which interact with TLR receptors. Therefore, the authors emphasize that HMGB1 has dual activity. It is speculated that this serves our body’s need to sacrifice or reconstitute tissue depending on whether an inflammatory stimulus is present or not [24]. Here we have shown that HMGB1, which is released during aseptic injury, is involved in preparing immune cells for further contact with pathogens. Therefore, a promising role for HMGB1 protein as a marker of inflammation and as a novel therapeutic target is evident.