Introduction
Inflammatory bowel disease (IBD) is a group of diseases characterized by refractory and chronic inflammation of the bowel, mainly including ulcerative colitis (UC) and Crohn’s disease (CD) [1]. Over the past few decades, epidemiological studies have shown that the incidence and prevalence of IBD have stabilized in North America and Europe but have been rising rapidly in Asia as well as some developed and developing countries in other areas [1–3]. Thus, IBD has become a global health issue.
There are some conventional therapies in clinical practice among patients with IBD, including 5-aminosalicylic acid derivatives, corticosteroids, and immunosuppressants. However, in patients with moderate to severe disease and with poor efficacy of the above therapies, biologics, especially anti-tumor necrosis factor α (TNF-α) agents, have been widely used [4, 5]. Anti-TNF-α agents play an anti-inflammatory role by specifically blocking the binding of TNF-α to receptors and inhibiting the production of interferon γ (IFN-γ) in the colon and stimulated T lymphocytes [6], which could effectively relieve intestinal inflammation and reduce hospitalization and surgery rates in patients with IBD. Under certain circumstances, anti-TNF-α agents are recommended as first-line biologic therapy [7].
Helicobacter pylori is a Gram-negative bacterium colonizing the gastric mucosa, which can cause chronic inflammation and even lead to gastric cancer [8]. After adapting to the acidic environment of the gastric mucosa, H. pylori uses pili to cross the mucus layer and attach to host cells by adhesins. H. pylori can secrete virulence factors including vacuolating cytotoxin A (VacA), cytotoxin-associated gene A (CagA), urease and catalase, thereby interfering with host metabolic pathways and inducing immunologic damage to gastric mucosal cells [9]. Additionally, some research suggests that chronic H. pylori infection may have a negative association with IBD [8, 10]. As an area with high prevalence of H. pylori, Asia has a relatively low incidence and prevalence of IBD. Nevertheless, the incidence of IBD has shown an increasing trend in some newly industrialized areas in East Asia, while the rate of H. pylori infection has been gradually decreasing [11].
There are few studies discussing the effect of H. pylori infection on the treatment of IBD, and it is inconclusive whether the presence of H. pylori affects the effectiveness of IBD treatment, especially in patients taking biologics. The aim of this review is to summarize the potential mechanisms of H. pylori and anti-TNF-α agents in the development and treatment of IBD, and to explore the potential interaction between H. pylori infection and anti-TNF-α agents.
Pathogenesis of inflammatory bowel disease
Previous studies suggest that IBD might be related to genetics, intestinal microecology, and immune imbalance, yet the etiology and pathogenesis still remain unclear [12, 13].
Family history is the strongest independent risk factor for IBD. Numerous studies have demonstrated that the risk of IBD is significantly higher among relatives of IBD patients, and this phenomenon is more pronounced in CD [14]. In recent years, scientists have been working on identifying IBD susceptibility loci through genome-wide searches to find the genes that contribute to disease susceptibility. Up to now, more than 200 gene loci have been identified as associated with UC and CD, some of which overlap between the two diseases [12, 13]. However, not everyone who carries the IBD-associated genes will develop the disease, since the gut microbiota also plays an important role.
Studies have shown differences in the diversity and abundance of microbial species in the gut or feces between IBD patients and healthy individuals [15]. For example, Faecalibacterium prausnitzii, which generates butyrate and ameliorates colorectal colitis, is significantly reduced in patients with IBD patients [15, 16]. However, Escherichia, specifically Escherichia coli, as a potentially harmful microbiota that promotes inflammatory responses, increases in abundance [15, 17, 18]. The hypothesis that intestinal microbiota plays an important role in the development of IBD has been supported by the successful clinical remission of IBD patients undergoing fecal microbiota transplantation, which is an emerging treatment [19].
Immune disorders are considered to be the major mechanism of IBD, which can be divided into innate immune disorders and adaptive immune disorders. Immune disorders in IBD are characterized by (1) epithelial damage, (2) inflammatory expansion driven by intestinal flora and massive cellular infiltration, including lymphocytes and dendritic cells (DCs), into the lamina propria, and (3) the failure of immune regulation to control the inflammatory response [12].
Innate immunity mainly involves neutrophils, DCs, macrophages, and natural killer (NK) cells. Neutrophils produce high levels of reactive oxygen species, which damage the epithelial barrier and encourage inflammatory reactions. Moreover, neutrophils produce pro-inflammatory cytokines such as interleukin (IL)-1β, IL-8, TNF-α and leukotriene B4, thereby attracting monocytes and more neutrophils to participate in the inflammatory sites [20]. Immature DCs release pro-inflammatory cytokines including IL-6, IL-12, IL-18, and IL-23, mediating the helper T (Th) 1 response and leading to local intestinal inflammation [20, 21]. Macrophages increase the expressions of pro-inflammatory factors including TNF-α, IL-6 and IL-β, and secrete IL-10 to maintain intestinal homeostasis, thereby controlling the progression of IBD [20]. Cytokines can activate NK cells, causing them to release huge amounts of hazardous particles and trigger apoptosis [21].
The inflammatory response in IBD is mostly mediated by adaptive immunity [22]. A study found that UC is related to Th2 reaction, while CD has more pronounced Th1 and Th17 reactions [23]. Th1 is induced by IL-12 and IL-18 to release IFN-γ and IL-2. Th2 plays a protective role and activates B lymphocytes by producing IL-4, IL-5 and IL-13. Th17 mainly secretes IL-17A to mediate inflammation [21, 24]. In IBD patients regulatory T (Treg) cells malfunction, which inhibits the release of IL-10. At the onset of the inflammatory response, the axis of Th17 and Treg is tilted in favor of the Th17 response [21].
Mechanisms of anti-TNF-α agents in inflammatory bowel disease
Biologics are widely applied in the treatment of IBD, among which anti-TNF-α agents are most commonly used in clinical practice. Drugs targeting TNF-α, such as infliximab, adalimumab, and certolizumab, have been proven efficacious and approved for the treatment of IBD in many countries [5].
The key pharmacological effects of anti-TNF-α agents are neutralizing sTNF-α and blocking mTNF-α [25]. STNF-α binds to TNFR1, and high levels of ubiquitinated RIP1 contribute to the formation of complex I, which activates the NF-κB and JNK signaling pathways and allows cell survival. If RIP1 is not ubiquitinated, complex II is formed, which activates the caspase pathway and leads to apoptosis. However, mTNF-α binds not only to TNFR1 but also to TNFR2, recruits TRAF and cIAP1/2, activates the non-classical NF-κB pathway, and promotes cell survival [6, 26, 27].
Strong evidence indicates that there are additional mechanisms of action of anti-TNF-α agents in IBD because mere neutralization of sTNF-α is insufficient to ameliorate chronic intestinal inflammation [25]. Once bound to mTNF-α, anti-TNF-α agents such as infliximab can inhibit cytokine production by monocytes, macrophages, and T lymphocytes and encourage apoptosis or cell lysis. It has been found that anti-TNF-α agents could reduce the secretion of TNF-α, IL-1, IL-6, and IL-8 by lipopolysaccharide (LPS)-stimulated monocytes in vitro [28], and activated the Fc region to differentiate monocytes into regulatory macrophages, thereby inhibiting T lymphocyte proliferation and producing IL-10 [29].
T lymphocytes play an important role in the pathogenesis of IBD, and anti-TNF-α agents may modulate the immune response in IBD patients by affecting T cell subsets. The decrease of pro-inflammatory T cell subsets (such as Th1, Th2, or Th17 cells) inhibit CD4+ and CD8+ T cell activation and decrease the secretion of pro-inflammatory cytokines (including IFN-γ, IL-13, IL-17A and TNF) and granzyme A. An increase in anti-inflammatory T cell subsets results in increased expression levels of CD4+CD25+Foxp3+ and CD4+CD25-Foxp3+ Treg and a general reduction of Treg cells in the mucosa. A number of experiments have shown that GM-CSF secretion by T lymphocytes is inhibited by infliximab, which leads to reduction of the number of peripheral blood neutrophils and lamina propria polymorphonuclear cells and decreased secretion of pro-inflammatory cytokines and chemokines, and consequently achieves the effect of suppressing intestinal inflammation. In addition, anti-TNF-α agents not only inhibit CD4+ T cell activation but also down-regulate IL-21 expression in mucosal CD4+ T cells, thereby inhibiting Th17 differentiation [28].
Other significant pathways for anti-TNF-α agents in IBD include antibody-dependent cytotoxicity and complement-dependent cytotoxicity. After the antibody binds to target cells, the Fc structural domain is recognized by the Fc receptor of effector immune cells (NK cells) that release cytotoxic proteins (e.g. perforin and granzyme), which subsequently leads to target cell lysis. Antibody binding to target cells can also lead to complement activation, which consists of several different proteins that react as a cascade to eventually form a membrane attack complex that induces cell death [28].
Protective effect of H. pylori infection on inflammatory bowel disease
Epidemiological studies and animal experiments generally support a negative association between H. pylori and IBD, indicating that H. pylori has a specific protective effect on IBD [10, 30-31]. According to a meta-analysis, H. pylori infection is one of the factors lowering the risk of IBD [32]. In addition, the eradication of H. pylori may lead to the recurrence of IBD [33].
Some researchers believe that the reduced gastric acid concentration and hypergastrinemia caused by H. pylori can alter the intestinal flora and achieve a new physiological balance [11], thus protecting patients from IBD. Previous studies indicated that the ratio of Firmicutes/Bacteroidetes had significantly decreased in H. pylori-positive patients [34], and the number of Bifidobacteriaceae increased significantly after successful eradication [35]. Eradication of H. pylori destroys the balance of the intestinal flora and results in the microbiota composition of IBD [11], which may be one of the reasons for the relapse of IBD.
Recent studies have pointed to the possible involvement of CagA in the protective mechanisms of IBD. There is evidence of a significant association among CagA seropositivity, H. pylori exposure and reduced odds of IBD (especially CD), and that CagA seropositivity has a protective effect on IBD in susceptible populations [36].
H. pylori-induced immunomodulation is an important defense mechanism. 1) The NF-κB pathway is ineffectively activated by the unique structure and poor bioactivity of H. pylori LPS, which prevents the release of pro-inflammatory cytokines including TNF-α, IL-1β, IL-6, IL-12, IL-17, IL-23 and IFN-γ [37]. 2) H. pylori activates autophagy genes (NOD2 and ATG16L1) that express autophagosome, and inhibit MHC II expression and the NF-κB pathway. The imbalance between inflammation and autophagy may play a crucial role in inducing the formation of semi-mature DCs. Semi-mature DCs express high levels of transforming growth factor β (TGF-β), IL-10 and IL-18, which induce differentiation of Treg and inhibit the conversion of naive Th0 cells to Th1 and Th17 cells, thereby reducing the production of pro-inflammatory cytokines [11, 37-39]. 3) High levels of CD4+CD25+Foxp3+ expression polarize Treg-dominated adaptive immunity, suppress effector T cell activity, and maintain immune system homeostasis [37, 39, 40]. 4) H. pylori can also activate a trimer composed of NOD2, P38 and hnRNP-A1, which subsequently enters the nucleus to stimulate transcription of IL-10. Interleukin 10 and TGF-β are responsible for activating the Smad signaling pathway and downstream protective mechanisms, inhibiting the NF-κB signaling pathway and Toll-like receptor (TLR) expression, and inducing production of CDX2 and transcription of MUC2 [37, 39]. 5) Binding of H. pylori to TLR2/NOD2 activates NLRP3 and caspase-1, promotes IL-18 secretion, and induces Treg differentiation. Since the production of pro-IL-18 induced by LPS through NLRP3 is not dependent on the NF-κB pathway, pro-IL-18 can be stably expressed in the cytoplasm [37, 39].
It has been discovered that H. pylori has a more significant protective effect against CD compared to UC, which may be explained by the fact that CD is linked to a Th1-driven immune response while UC is caused by a Th2-driven one, and the primary T-cell response induced by H. pylori is a Th1-mediated one [38].
H. pylori eradication therapy may increase the risk of IBD. In addition to altered intestinal flora, Yu et al. [37] proposed an immunomodulatory hypothesis that the balance of Th1- and Th2-driven immune responses is disrupted after H. pylori eradication, and a polarized Th1 may favor the development of IBD in some genetically susceptible individuals. Research on the relationship between IBD and the eradication of H. pylori and mechanisms involved is still needed.
Pathways co-influenced by H. pylori and anti-TNF-α agents
There is no study discussing the interaction between H. pylori and anti-TNF-α agents. Abd et al. [41] assessed the probabilities of amelioration of IBD symptoms based on H. pylori infection status and IBD treatment options, but did not obtain significant results. We compared the immune-related mechanisms of action of H. pylori and anti-TNF-α agents in IBD and found that they affect the same pathways, involving the activation and inhibition of T lymphocytes and cytokines. We summarize the possible mechanisms of the interaction between them in IBD (see Table 1 and Figure 1).
Fig. 1
Possible mechanisms of interaction between H. pylori and anti-TNF-α agents in inflammatory bowel disease. TNF – tumor necrosis factor, LPS – lipopolysaccharide, IL – interleukin, Th – helper T, IFN – interferon, Treg – regulatory T, TGF – transforming growth factor
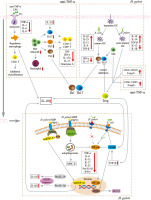
Table 1
Interaction and signaling pathways concerning the crosstalk between H. pylori and anti-TNF-α agents in inflammatory bowel disease
Effect on T lymphocytes
The anti-inflammatory T cell subpopulation (Treg) and the pro-inflammatory T cell subpopulation (Th1, Th17) are jointly affected by H. pylori and anti-TNF-α agents in IBD. H. pylori induces the formation of tolerant semi-mature DCs and activates hnRNP-A1, NLRP3 and caspase-1. Then H. pylori promotes TGF-β, IL-10 and IL-18 secretion, and induces Treg differentiation, thereby inhibiting the conversion of immature Th0 cells to Th1 and Th17 cells [11, 37, 38, 40]. Anti-TNF-α agents redistribute Treg, reduce pro-inflammatory T cell subsets (such as Th1, Th2 or Th17 cells), and inhibit CD4+ and CD8+ T cell activation [28]. Both H. pylori and anti-TNF-α agents lead to high levels of CD4+CD25+Foxp3+ expression, activation of Treg, and suppression of effector T cell activity [11, 28, 37, 38, 40].
Foxp3, a marker of CD4/CD25 Treg, is a major regulator of Treg [42, 43]. Foxp3 and inflammatory TLR signaling control the proliferative and repressive functions of Treg by balancing the metabolism between glycolysis and oxidative phosphorylation [42]. CD4/CD25 T cells transduced with Foxp3 up-regulate the expression of Treg-related molecules. It has been shown that CD25/Foxp3 T cells are engaged in the immune response to H. pylori and control the degree of H. pylori-induced immunopathology [43]. Anti-TNF-α agents such as infliximab have been found to restore Foxp3 expression that is reduced in patients with autoimmune disorders, and to down-regulate the suppressive role of Treg [27]. The suppressive function of Treg has an irreplaceable role in preventing target organ damage caused by autoimmune and inflammatory responses. Treg can enhance suppressive function through specific effector pathways or responses, including contact-dependent mechanisms, immunomodulatory cytokines such as IL-10 and TGF-β, and influencing the metabolism of target cells. Treg also has a Th cell transcriptional mechanism that can modulate the inflammatory response by controlling Th cells [44] and inhibiting the release of pro-inflammatory cytokines.
Effect on cytokines
Both H. pylori and anti-TNF-α agents in IBD promote the secretion of the anti-inflammatory cytokine IL-10 and inhibit secretion of the pro-inflammatory cytokines including TNF-α, IL-1β, IL-6, IL-17A and IFN-γ. H. pylori stimulates IL-10 transcription by inducing tolerant semi-mature DCs and activating hnRNP-A1 [11, 37-40]. Anti-TNF-α agents activate the Fc region to differentiate monocytes into regulatory macrophages, inhibit T lymphocyte proliferation, and produce IL-10 [29]. IL-10 activates the Smad signaling pathway and downstream protective mechanisms, inhibits the NF-κB signaling pathway, induces Treg differentiation, and suppresses the secretion of pro-inflammatory cytokines including TNF-α, IL-1β, IL-6, IL-17A and IFN-γ [37].
IL-10 is an immunomodulatory factor associated with the development of IBD and Treg-regulated intestinal homeostasis [37, 45]. Studies on both animals and people have shown that IL-10 or its receptor gene disruption causes spontaneous IBD. The effect of IL-10 on IBD is dependent on IL-10 signaling in macrophages, which is required for anti-TNF-α agents induced polarization of macrophages to a regulatory M2-like phenotype. Blocking IL-10 signaling pharmacologically with IL-10Rα-blocking anti-bodies decreases the therapeutic efficacy of anti-TNF-α agents [46]. A clinical study found that, compared to H. pylori-negative patients, H. pylori-positive patients with moderate-to-severe active UC have higher levels of IL-10, which may be explained by the mechanism that IL-10 is triggered as a compensatory mechanism during H. pylori colonization, building tolerance and reducing disease pro-inflammatory activity through Treg [39].
TNF-α is a homologous trimer protein consisting of 157 amino acids and is mainly produced by activated T lymphocytes, macrophages, and NK cells [6]. Aberrant regulation of TNF-α is associated with IBD and H. pylori infection [47, 48]. H. pylori-induced TNF-α secretion is reduced in cells with homozygosity of G alleles [49]. In contrast, TNF-α concentrations in the feces of CD patients were significantly higher and correlated with disease activity, which may be related to the stimulation of Th1 cell responses that produce large amounts of IL-2, IFN-γ, and TNF-α. TNF-α itself plays a positive feedback role in TNF-α secretion, and anti-TNF-α treatment inhibits the expansion of aggressive T lymphocytes and reduces TNF-α secretion [48]. The TNF signaling pathway is a mutual pathway among IBD, H. pylori, and anti-TNF-α agents, suggesting that H. pylori infection and anti-TNF-α agents may have synergistic effects in IBD. Further clinical experimental studies need to be conducted in the future.
Discussion and Outlook
Previous studies suggest that both H. pylori infection and anti-TNF-α agent treatment have a positive effect on patients with IBD patients. Moreover, there is evidence of pathways co-influenced by H. pylori and anti-TNF-α agents, which might be the underlying mechanisms in affecting IBD. The authors came to the conclusion that in patients with IBD who receive biologics treatment and are H. pylori-positive, H. pylori eradication may not be done immediately. Since the infection status of H. pylori is not routinely tested in patients who receive biologics in clinical practice, it has not been reported concerning the interaction between H. pylori infection and anti-TNF-α agents in patients with IBD. A study found that the application of anti-TNF-α agents lowered the incidence of H. pylori infection, yet the result was not statistically significant [50]. Therefore, further studies are needed to explore the interaction of H. pylori infection and anti-TNF-α agents in patients with IBD as well as its mechanisms.
We suggest that multi-center clinical trials should be conducted to confirm the association between H. pylori and anti-TNF-α agents as well as their interaction in the treatment of IBD. Furthermore, experimental studies in vivo and in vitro are required to explore the mechanisms of the crosstalk between them. The results would contribute to the future development of the treatment of IBD and the application of biologics.