Introduction
The first analysis of 621 liver resections in patients with colorectal cancer (CRC) from a multicentre study was published in 1977, and postoperative mortality for major liver surgery ranged from 13–20%, with intraoperative haemorrhage as the main causative factor [1]. In the 1990s the average rate of postoperative mortality decreased to 5% and even less in specialized high-volume centres [2]. Consequently, the number of liver resections performed worldwide increased in geometrical progression. Such an evolutional leap occurred due to improved understanding of both surgical and oncological principles of treatment of primary and secondary liver malignancies.
However, each type of liver resection is accompanied by trauma, surgical stress, and aseptic inflammation. As well as immediate damage during surgery, remnant liver tissue undergoes delayed, secondary damage by proinflammatory cytokines and dysregulated oxidative stress with tissue respiration alterations by radical oxygen species (ROS) and its derivates. Strategies of blood loss prevention during liver resection in most cases involve control of blood inflow Pringle manoeuvre (PM) or a combination of restriction of inflow and outflow (total vascular exclusion). Basically, the resection of metastatic lesions in the liver’s “right venous core” correlates with a longer period of the organ remaining in the conditions of PM and inducing pathologic ischaemia-reperfusion injury (I/R) [3, 4]. Most of the pathological changes that occur in hepatocytes under anoxic conditions are found particularly in the mitochondria. Dysfunction of O2 metabolism in the mitochondria leads to electron transport and mitochondrial respiration disorders. Under such conditions, there is a sharp decrease of pyrimidine nucleotide production, which in turn causes an increase of intracellular nicotinamide adenine dinucleotide phosphate (NADH\NAD+) level accumulation. Changes in oxidative phosphorylation processes lead to the activation of intracellular ATP depletion, acceleration of glycolysis, increase of lactate levels, and the effect of H+, Na+, and Ca2+ homeostasis. The above-described pathological changes of resected liver lead to severe disorders of hepatocyte function and liver in general. In turn, reperfusion causes cell damage by products of incomplete reduction of O2 by superoxide radicals (SR) during repeated inflow of O2 into ischaemic tissues. SR are produced both in cells (mitochondria) and in extracellular sources (NOX, iNOS neutrophils, platelets, lymphocytes). The inability to overcome the biological consequences of an I/R phenomenon among cancer patients has remained one of the main surgical issues throughout the last 3 decades [5–7]. Ischaemia-reperfusion deteriorative effect is mainly presented by triggering redox homeostasis imbalance, maintaining the process of sterile inflammation and possible mediating growth of “minimal residual disease” [6–8].
The theory of “oxidative stress”, which includes redox homeostasis and redox adaptation, was introduced more than 30 years ago [7]. It is well known that accurate control of the cell redox state is mandatory for maintaining structural integrity and physiological functions. The violation of redox homeostasis leads to various types of diseases, such as atherosclerosis, diabetes, neurodegeneration, and the initiation of tumour growth, including CRC [8]. The development of intracellular oxidative stress is promoted by SR and nitric oxide (NO), which directly affect intracellular respiration pathways by changing the mitochondrial redox state [9, 10]. The levels of mitochondrial NADH/NAD+ decreases, whereas the SR generation increases in response to the flavine and complex I with oxygen reduction through the ROS activation. Radical oxygen species derivatives (hydrogen peroxide and hydroxyl radicals or SR) le d to hepatocyte disfunction and injury [11]. That is why ROS and their derivatives are considered to be the main effectors of I/R toxicity [12].
Within the adenocarcinoma cells, high levels of SR formation can cause increased metabolic activity, mitochondrial dysfunction, peroxisome activity, increased redox-dependent cell signalling, oncogenic activity, increased oxidase activity of cyclooxygenase, lipoxygenase, and thymidine phosphorylase [13]. It has been proven in vivo that liver surgery in terms of PM is able to activate molecular pathways of cancer cell proliferation as part of the liver parenchyma regeneration process [14, 15]. This can be a potential cause of “dormant” tumour cell activation even during chemotherapy [16]. Although the exact mechanisms of CRC cell “dormancy” are poorly understood, it is known that changes in the redox state and SR generation in mitochondria of malignant and immune cells may initiate their rapid proliferation as well [17].
That is why a more profound understanding of the mitochondrial redox state in CRC patients’ liver surgery is necessary as an attempt to improve surgical and oncological treatment outcomes.
Material and methods
The aim of this study was to assess the values of molecular markers of the mitochondrial redox state of hepatocytes in CRC patients after liver surgery.
Between March 2017 and December 2020, 114 CRC patients were included in this prospective study. Patients underwent hepatic resections in the National Cancer Institute, (Kyiv). Preoperative characteristics are detailed in the Table 1. The inclusion criterion was CRC patients with ≥ 1 liver metastases, considered resectable (possibility of ≥ 30% parenchyma preservation). Exclusion criteria were patients who had more than 3 lung metastases, and/or peritoneal carcinomatosis. Primary outcomes were the changes of values of the redox state markers (SR, NO-Fe-S cluster proteins complexes, lactate, bilirubin [BL] and aminotransferases) during PM.
Table 1
Preoperative characteristics
The median age of the participants was 60 years, and the distribution by sex showed 66.6% males and 44.4% females. Most patients had primary tumours with depth of invasion of T3 (–59.6%) and without regional lymph node involvement pN0 (50.9%). Metachronous liver metastases were detected in 83.4%. Simultaneous colorectal and liver resections were performed in 12.3%. Morphological data showed liver margin status R0 and R1v in 94.7% and 5.3%, respectively. The median number of chemotherapy cycles before resection was 3. ASA scale status of patients undergoing surgery was 90.4% and 9.6% for ASA-2 and ASA-3, respectively (Table 1).
The PM was used in 59.6% of patients. Major liver resections (≥ 3 anatomical segment) were performed in 13.2%, while 86.8% of surgical procedures were done in a parenchyma sparing way. Tumour spread was evaluated by calculating the qualitative and quantitative characteristics of metastatic lesions and by semi-automatic volumetry. The median volume of resected metastases and number of resected metastases were 259 cm3 and 6 lesions, respectively. Colorectal cancer patients with 1, 2–5, 6–10, 11–15, and ≥ 15 liver metastatic lesions were detected in 16.6%, 48.3%, 21.1%, 10.5%, and 3.5% cases, respectively.
Preoperative management
All patients had histologically proven adenocarcinoma and were staged according to TNM 8th ed., serum carcinoembryonic antigen levels, total colonoscopy, thoracoabdominal computed tomography and abdominal magnetic resonance imaging had been performed systematically.
Surgical management
The surgical technique included crash-clamping principles, routinely adhered ≥ 1 mm minimal resection margin. In the case of metastatic lesion contact with main vascular liver structures of 1–2 order and absence of true invasion, a vascular detachment tactic was applied if possible [18]. All surgical procedures were accompanied by intraoperative ultrasound guidance. In order to control blood loss during liver parenchymal transection, we routinely applied an intermittent (20 min – ischaemia, 5 min – reperfusion) PM in its classical performance.
Assessed molecular markers
We evaluated generally accepted molecular markers of cytolysis and detoxification function of hepatocytes alanine aminotransferase (ALT), BL. By detecting the impact of the pathologic effect of I/R injury on electron transport in mitochondria respiratory chain complexes the NO levels with Fe-S cluster proteins had been investigated. The degree of hypoxia and the rate of toxic injury were estimated by the levels of serum lactate (within 24 hours of surgery) and in liver parenchyma (within 1 hour of completed transection). The SR generation speed was measured directly in the liver parenchyma. Tissue sampling was performed immediately after specimen removal, liver stump parenchyma at a distance 5 cm from metastatic lesions was extracted in 0.5 cm3 volume.
Qualitative and quantitative changes in the functioning of electron transport complex (ETC) were measured according to the positioning of electron paramagnetic resonance (EPR) signals (g-factor or spectroscopic splitting factor), also amplitude measurement of the corresponding signal in control and test tissue samples was performed. The state of FeS-proteins in the first mitochondrial ETC was studied (g-factor 1.94); dynamics of forming NO with FeS-protein complexes in mitochondrial ETC N-type (g-factor 2.03); dynamics of forming structural changes in mitochondrial ETC according to the triplet signal generation (g-factor 2.007). The speed levels of SR generation by NAD(P)H oxidase were registered by EPR method with the use of a spin catcher (2, 2, 6, 6-tetrametyl-4 oxypiperidine) (Sigma, USA).
Statistical analysis
A t-test was used to compare quantitative variables between groups if the distribution was parametric; ANOVA followed by the post hoc test and nonparametric test (U Mann-Whitney) were used to test the significance of differences. Statistical significance was determined as p < 0.05. Values are expressed as median ± min. and max. Multiple linear regression was done for the assessment of connections between the level of molecular markers and ischaemia duration. Statistical analyses were performed using Prism 8.0.
Results
Postoperative severe morbidity (grade IIIa and higher) was registered in 19 (16.6%) patients, and the level of hepatospecific complications was 7 (6.2%) (Table 2). Among severe hepatospecific complications, biloma and biliary fistula were the most reported. The median surgery duration was 306 (41–520) min., and perioperative blood transfusion was 0 (0–4) units. The median duration of warm ischaemia during PM liver parenchyma transection was (39 ± 25.8) minutes (Table 2).
Table 2
Operative and perioperative characteristics
Thirty-day postoperative mortality amounted to 1 (0.8%) case, which was caused by mesenteric thrombosis on the 5th postoperative day.
Alanine aminotransferase and BL levels preoperatively remained at 34.5 ± 14.9 IU/L and 12.4 ± 5.2 µmol/L respectively. The dynamics of total BL in blood changed from the moment of parenchymal transection on the 1st, 3rd, and 5th postoperative day (POD), respectively: 18.4 ± 5.8 µmol/L; 15.6 ± 5.4 µmol/L; and 12.5 ± 4.4 µmol/L. Alanine aminotransferase levels in blood plasma on the 1st, 3rd, and 5th POD were 178.8 ± 151.5 IU/L, 135.3 ± 111.7 IU/L, and 75.6 ± 68.8 IU/L, respectively (Fig. 1).
Fig. 1
Alanine aminotransferase and bilirubin levels dynamics on the 0, 1st, 3rd, and 5th postoperative day
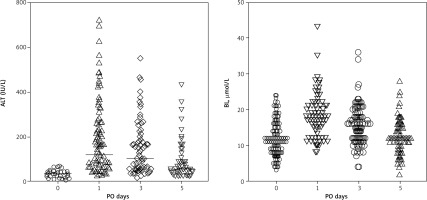
Serum lactate level in liver stump parenchyma within 1 hour of liver resection was 2.1 ± 0.4 RU whereas venous blood lactate level on 1 POD after the end of liver transection was 1.8 ± 0.6 mmol/L.
Mitochondrial redox state of adenocarcinoma cells in metastatic tissue and hepatocytes of adjusted parenchyma and liver stump were measured with the SR speed generation in CRC patients. Superoxide radical generation speed in metastatic tissue, within 1 cm of surrounding tissue and liver stump parenchyma at a distance 5 cm from metastatic lesions, was estimated, respectively, at 2.32 ± 0.19; 1.09 ± 0.1, and 0.36 ± 0.03 nmol/gm raw tissue • min (physiological condition value of SR generation speed in liver parenchyma is 0.22 ± 0.03 nmol/gm raw tissue • min). Pringle manoeuvre application was associated with higher SR level compared to liver surgery without blood inflow control 0.32 ± 0.12 and 0.42 ± 0.21 nmol/gm raw tissue • min, respectively (Fig. 2).
Fig. 2
The mitochondrial superoxide radical generation speed
Metastatic tissue – adenocarcinoma cells of colorectal cancer (CRC) liver metastatic lesions, adjusted – hepatocytes of adjusted parenchyma within 1 cm to CRC metastatic lesions, liver stump – stump liver parenchyma at a distance 5 cm from metastatic lesions, Liver stump with/without MP – stump liver parenchyma at a distance 5 cm from metastatic lesions stratified by factor of MP use
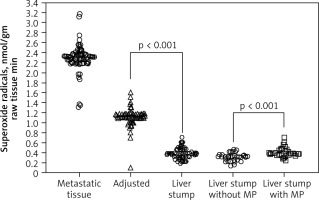
Decrease of mitochondrial ETC activity occurs as a result of forming NO with Fe-S cluster protein complexes (g = 2.03), the level of which increases. The levels of NO-Fe-S cluster protein complexes in liver stump parenchyma in the end of transection with and without PM were 0.35 ± 0.09 RU and 0.16 ± 0.04 RU, respectively.
In the performed study we conducted multiple linear regression analysis concerning the assessment of associative connection between the durability of applied liver ischaemia and levels of studied molecular markers (Table 3).
Table 3
Multiple linear regression for assessment of connections between the level of molecular markers and ischaemia duration
The result of multiple linear regression analysis showed the presence of a moderate negative connection according to the intercept: –74.2 ± 10.8 (95% CI: –95.6 to –52.5) p < 0.001, R-squared = 0.73. Moreover, the result of multiple linear regression analysis showed the presence of a persistent positive connection between hepatic ischaemia during PM and the assessed molecular markers. It was shown that there is a positive associative connection between the ischaemia and BL level on the 3rd day PO: 0.62 ± 0.3 (95% CI: 0.11–1.12) R2 = 0.012, p = 0.02. Also, long-term ischaemia reliably increased the ALT level (3 POD): 0.06 ± 0.02 (95% CI: 0.03–0.099), R2 = 0.53, p = 0.006. In doing so, the most significant impact of long-term ischaemia was found regarding the rate of SR generation speed in liver stump: 152.4 ± 24.4 (95% CI: 104.1–200.7), R2 = 0.46, p = 0.001.
Discussion
A proper liver resection strategy is the cornerstone of CRC with liver metastases treatment (complete removal of metastatic lesions allows an overall survival rate of 43–65%) [19]. Currently, individualized CRC prognosis is the subject of active research. It is known that surgical stress is a powerful impulse to liver regeneration, regulation of its volume and growth, as well as atrophy. In turn, warm I/R injury that occurs during liver resection potentiates the effect of resection stress and stimulates the generation of the SR, which activates the signalling cascades of liver regeneration. The mean duration of liver warm ischaemia (39 ± 25.8 min.) in this study was within the permissible norm, which is 90–120 min [20]. Our analysis demonstrated mild negative effects of used ischaemia duration as cytolysis and hyperbilirubinemia during 3–5 POD. Even though there was no hyperbilirubinaemia after the 5th POD in the cohort of patients with PM application that demonstrates low risks of the liver failure. We also note that in this study the principles of parenchyma preserving liver surgery were implemented, and the cohort of major liver resections did not exceed 13%. Thus, we had no risk of parenchymal deficiency in the postoperative period, and all procedures were planned carefully. Furthermore, we confirmed the data that liver resection with PM leads to reprogramming of mitochondrial metabolism from oxidative phosphorylation to glycolysis. Cellular hypoxia was detected immediately from the liver surgery completion with the high level of liver tissue lactate and 1st POD. It was found that the adenocarcinoma tissue of metastatic lesions and the surrounding liver are characterized by activation of sources of redox molecule generation, in particular SR, compared to the same indicators in normal liver stump.
Moreover, we identified that the hypoxia deepening leads to a significant increase of the SR generation rate. It is proven in the literature that the high SR activity changes the intercellular matrix rheology due to the activation of the matrix metalloproteinases enzymes group [21]. The activities of these hypoxia-dependent enzymes at high levels are also recorded during tumour growth and metastatic spread of the CRC [22]. Furthermore, data from this study has shown the increasing of NO-Fe-S cluster protein levels in liver stump parenchyma after the end of the liver surgery in the MP cohort patients. NO-Fe-S cluster proteins are responsible for numerous functions in the mitochondrial respiratory chain, including the synthesis of O2 and free radicals, and the regulation of matrix metalloproteinases.
During the past 5 years there has been a huge increase in the pool of experimental and clinical confirmation of the possibility of the pathological influence of surgical interventions on the activation of “dormant” micrometastases in patients with CRC [23]. The theory of increased risk of recurrence or oncologic disease progression after resection has been frequently found in the literature for a long time [24], but it still does not have an adequate explanation or justification. We studied this phenomenon from the point of view of mitochondrial ETC functioning in hepatocytes from different liver sites and found the dependence of SR levels from the duration of ischaemia, which indirectly confirms the negative potential of I/R injury in oncologic patients. Of great concern, the insufficient understanding of biological pathways of malignant growth and tumour progression is the greatest “stumbling block” on the path to successful CRC treatment. And SR research as a prognostic factor is crucial in the attempt to personalize surgical treatment of patients with CRC liver metastases.
Conclusions
Ischaemia-reperfusion injury and Pringle manoeuvre exacerbates the disruption mitochondrial respiratory chain and potentiates SR generation. The redox status molecular markers of the hepatocytes in CRC patients with liver metastases can be used to assess the functional status of organs and tissues and improve the existing surgical strategies.