Purpose
Concurrent chemoradiation is considered the standard treatment for locally advanced cervical cancer [1]. Depending on the case, external beam radiation therapy (EBRT) alone, brachytherapy followed by EBRT, or combination chemotherapy can be employed. The combination of radiation therapy and chemotherapy is primarily used for tumors larger than 4 cm or clinical stage II or higher, with brachytherapy being an essential component after external irradiation [2, 3].
Intra-cavitary brachytherapy (ICBT) allows for precise delivery of radiation dose (Gy) near to the tumor. According to the inverse square law, the radiation dose (Gy) is inversely proportional to the square of distance from the source. This approach enables the administration of a high radiation dose (Gy) to the tumor while safeguarding surrounding healthy organs. ICBT remains the only established method capable of providing necessary high-dose (Gy) for effective control of cervical cancer without causing adverse effects [4].
Irradiation of organs at risk (OARs) surrounding the uterus can lead to intestinal inflammation, proctitis, and cystitis. Therefore, optimizing dose (Gy) to OARs in gynecological brachytherapy is crucial in minimizing the risk of complications, despite advantages of ICBT [5].
Multiple studies have investigated the effect of bladder filling on the absorbed dose (Gy) in the bladder, rectum, sigmoid colon, and small bowel [6-8]. In the literature, researchers have assumed the existence of an optimal bladder volume, and classified bladder volume into several groups. They have used a prospective method to analyze the dose to surrounding organs after artificially filling the bladder as well as a retrospective method to analyze previous data. Increasing bladder volume (BV) has been found to decrease the dose to the small bowel; however, different studies have employed varying criteria for BV (cm3). Consequently, establishing a consensus on the optimal BV (cm3) to minimize OARs doses has proven challenging.
Researchers have explored the anatomical relationship between uterine motion and surrounding organs [9-12]. The position and volume (cm3) of organs within pelvic cavity vary among individuals. However, most studies have failed to consider the effects of changes in bladder shape, resulting from individual anatomical displacement of the uterus, or alterations in its size and position due to tumor growth. If the impact of pushing the uterus upward is dependent upon the shape of bladder, it could influence the analysis of dose-volume histogram (DVH) when considering bladder volume. Consequently, it is necessary for researchers to analyze the relationship between the dose (Gy) administered to surrounding organs by subdividing BV (cm3) while accounting for bladder shape. The aim of the current study was to investigate the impact of bladder filling type (BFT) classification on optimizing BV (cm3) and its effects in reducing the dose (Gy) on OARs during cervical cancer brachytherapy.
Material and methods
Patients and treatment
This retrospective study was approved by the Institutional Review Board of Keimyung University, Dongsan Hospital (approval No.: DSMC 2023-01-070). In total, 269 patients with locally advanced cervical cancer who had undergone curative radiation therapy between January 2016 and March 2022 were selected. Relevant information were obtained from their medical records. Patients received a combination of brachytherapy and EBRT (prescription dose of 45-50.4 Gy, 1.8 Gy/fraction or < 60 Gy if lymph nodes were considered), along with high-dose-rate (HDR) 3D-optimized ICBT based on magnetic resonance imaging (MRI) (prescription dose of 30 Gy, 5 Gy/fraction). Cancer stage was determined according to the International Federation of Gynecology and Obstetrics (FIGO) 2013 and 2018 staging systems. Stages I, II, III, and IV were identified in 49 (18.2%), 118 (43.8%), 83 (30.9%), and 19 (7%) patients, respectively.
Patients were positioned supine on a lithotomy device. To minimize undesired anatomical deformities, a Foley catheter was inserted into the bladder for most patients, and 50 ml to 200 ml of normal saline was injected. An enema was administered to regulate the volume of bladder and rectum. Intravenous analgesia was applied before simulation and treatment to alleviate pain. Tandem and ring type applicator was inserted through the vagina into the uterus/cervix, followed by insertion of a rectal retractor or packing gauze to increase the area between the rectum and cervix.
Imaging and contouring
Pre-treatment MR images acquired for diagnostic purposes were used for the bladder morphology analysis. MR images obtained during treatment were taken after the applicator was inserted, with the patient being moved to MR imaging room on a mobile bed, and images were captured while the knees were in a relaxed position. Therefore, there was a difference between pre-treatment and intra-treatment MR images in terms of the presence or absence of the applicator. A simulation MRI was performed to outline the target and OARs. MRI scanning was conducted using a 3.0 Tesla (T) MR system (Magnetom Vidar, Siemens Medical Solutions, Erlangen, Germany). 2D T2-weighted images using a turbo spin echo MRI sequence were obtained with the following parameters: repetition time/echo time, 5,470/134 ms; slice thickness, 3.0 mm with a 4.02 mm spacing between slices; field of view, 260 × 260 mm; bandwidth, 248 Hz/pixel; flip angle, 120°; and matrix size, 448 × 269. T2-weighted images were acquired in paraxial plane perpendicular to the tandem tip, involving the fifth lumbar vertebra to the upper third of the femur. Gross target volume, high-risk clinical target volume (HR-CTV), intermediate-risk clinical target volume, and OARs (i.e., bladder, rectum, sigmoid colon, and small bowel) were delineated based on recommendations of the Gynecological Groupe Européen de Curietherapie-European Society for Radiotherapy & Oncology (GYN GEC-ESTRO) and the International Commission on Radiation Units and Measurements Report 89, using MRIs [13-16].
Treatment planning
3D-optimized ICBT was implemented using dose distribution obtained from treatment planning system (TPS), and the plan was optimized to meet the standards recommended by iso-dose (Gy) and GEC-ESTRO working groups (I, II, and IV). MRI-guided 3D ICBT plan was generated using Oncentra TPS (Elekta Brachytherapy Solutions, Stockholm, Sweden). Radiation source was iridium-192, with the source positioned at 2.5 mm intervals from the tip. Initially, auto-optimization was conducted to ensure that HR-CTV 90% received an EQD2 of over 90 Gy, followed by manual adjustments to align with permissible dose limits for normal organs. Prescribed doses (Gy) for HR-CTV were optimized through the separation between dwell positions, taking into consideration DVHs of the rectum, bladder, sigmoid colon, and small bowel. To calculate equivalent dose in 2 Gy fractions (EQD2), a linear quadratic model was applied, with an α/β value of 10 Gy for CTV and an α/β value of 3 Gy for organs at risk [14]. Treatment goals were to deliver a minimum of 90 Gy to D90 (EQD2) of HR-CTV, keep the dose to the bladder below 90 Gy, limit the dose to the rectum and sigmoid colon below 75 Gy, and restrict the dose to the small bowel below 60 Gy. The GEC-ESTRO working group for image-guided brachytherapy (IGBT) designated the maximum absorbed dose D2cc (Gy) value as a surrogate for “hot spot” of OARs. Radiation-induced injury was likely caused by a hot spot within the irradiated volume. D2cc (Gy) values for the bladder, rectum, sigmoid colon, and small bowel were obtained from DVHs [13, 15].
Analysis of bladder appearance and position change
Bladder filling type classification and uterine motility were analyzed using pre-treatment MRI as well as MRI simulation in picture archiving and communication systems. Bladder types were distinguished by superimposing bladder contours on MR images using Python code for analysis. BFT classification consisted of three types: tilted or T shape, curved or C shape, and E group (Figure 1). The tilted type was defined as a shape where either side of the bladder was no more than 90° or 270° in the para-axial plane, where the applicator ring was most visible. The curved type was defined as a shape where the left and right sides exceeded 90° and 270°, respectively, relative to the applicator ring, considering the tandem and applicator. Cases, which were not included in the tilted and curved shape groups were classified into the E group. Furthermore, BFT was divided into three sub-groups based on BV (cm3) as follows: BV < BV 300 cm3 (t3, c3, e3) (Tables 1, 3, 4). Bladder volumes were calculated from the contour data in Oncentra TPS. To analyze pelvic cavity, five anatomical position changes were defined, which could affect BFT: 1. The length from the center to the right border of the bladder (CRB) on axial MRIs; 2. The length from the center to the left border of the bladder (CLB) on axial MRIs; 3. The length from the central axis of the tandem to the mid-sagittal line on axial MRIs; 4. The length from the anterior fatty layer to the outer margin of the uterus (AU) on sagittal MRIs; and 5. The length from the posterior outer margin of the ureter to the inner margin of the sacral bone (PU) on sagittal MRIs (Figure 2).
Fig. 1
Examples of bladder type classification: 3D reconstruction images demonstrate the appearance of a representative bladder before and after intra-cavitary brachytherapy (ICR) applicator insertion, categorized into three different bladder types, i.e. tilted type (A, B), curved type (C, D), and E group (E, F). ICR applicator: tandem and ring is indicated by a circle with crossline
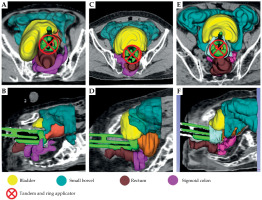
Fig. 2
Axial (A, B) and sagittal plane (C) images on T2 TSE MRI showing measurement locations for analysis
AU – anterior fatty layer to the outer margin of the uterus, PU – posterior outer margin of the ureter to the inner margin of the sacral bone
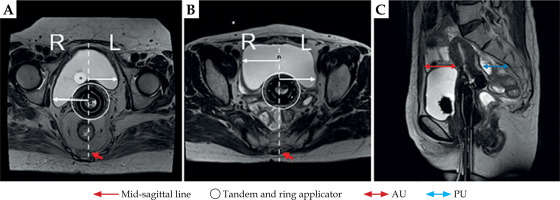
Table 1
Shapiro-Wilk normality test and group characteristic analysis results according to bladder shape consideration
Table 2
Mean of maximum dose irradiated to the bladder (D2cc), rectum (D2cc), small bowel (D2cc), and sigmoid colon (D2cc) between groups in classification results and Spearman’s rank correlation coefficient
Table 3
Results of the effect of changes in bladder filling volume and differences in left and right bladder lengths on bladder type classification before and during intra-cavitary brachytherapy (ICBT) simulation
Statistical analysis
Normal distribution of OAR dose was confirmed using Shapiro-Wilk (SW) test. Spearman’s rank correlation coefficient was employed to analyze the correlation between BV and OAR dose. To examine the impact of bladder type on center displacement, one-way analysis of variance (ANOVA) was conducted using the length difference between CRB and CLB. For assessing the homogeneity of population variances, Levene’s test was utilized. Games-Howell test was applied as a post-hoc analysis to evaluate differences among the groups. Additionally, Wilcoxon signed-rank test was applied to analyze the significance probability of a difference between the anterior and posterior spaces of the uterus (AU and PU) based on the BFT classification. Statistical significance was determined with an α level of 0.05, using SPSS version 17.0 (SPSS Statistics for Windows, Chicago, SPSS Inc., USA).
Results
The two distinctive bladder shapes were demonstrated by overlapping bladder contours: the tilted bladder and the curved bladder (Figure 3). All bladders that were excluded from these two bladder shapes were included into the E group. D2cc (Gy) of the bladder, rectum, sigmoid colon, and small bowel in DVH obtained from 3D-optimized ICBT plans for a total of 269 patients were investigated (Table 1). To determine whether there was a normal distribution of the maximum absorbed dose distribution in each organ with an increase in bladder volume (BV), Shapiro-Wilk (SW) test was utilized. Initially, when considering only BV without the shape of the bladder, SW test revealed a p-value of less than 0.01 for all organs, indicating that data did not follow a normal distribution. The mean BV was 263.5 cm3 when the bladder type was not considered.
Fig. 3
Classification of the bladder type by using an image interpolation method. Convolution of bladder segmentations on tilted type (A) and convolution of bladder segmentations on curved type (B)
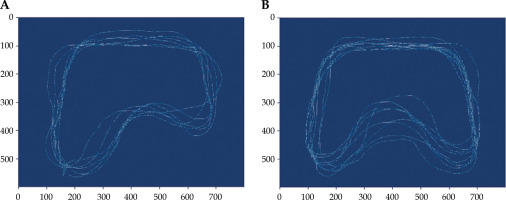
However, when both the BV and bladder shape were considered, SW tests showed 88 patients in the tilted group, 125 patients in the curved group, and 56 patients in the E group. In the tilted group, a p-value of > 0.05 indicated that the bladder and rectum fulfilled the condition of normality, while the sigmoid colon and small bowel showed a p-value of less than 0.05, indicating a deviation from normality. For the curved group, none of the organ data exhibited a normal distribution. In the E group, the bladder, when both the BV and bladder shape were considered, SW tests confirmed 88 patients in the tilted group, 125 patients in the curved group, and 56 patients in the E group. In the tilted group, a p-value of > 0.05 indicated that the bladder and rectum fulfilled the condition of normality, while the sigmoid colon and small bowel showed a p-value of less than 0.05, indicating a deviation from normality. For the curved group, none of the organ data exhibited a normal distribution. In the E group, the bladder and sigmoid colon data were found to deviate from a normal distribution. The difference in the mean BV between the sub-groups t2, c2, and e2 was within 2%.
When the bladder shape was not considered, the maximum absorbed dose of OAR did not follow a normal distribution. Consequently, non-parametric statistical method was employed to evaluate the correlation between the bladder volume (BV) and each OAR. Therefore, Spearman’s rank correlation coefficient test was performed. In the absence of bladder shape consideration, the correlations between the BV and maximum absorbed dose of the bladder and small bowel were found to be 0.266 and –0.287, respectively (Table 2). The D2cc (Gy) of the bladder increased with distension, while the D2cc (Gy) of the small bowel tended to decrease.
In the tilted group, the average D2cc (Gy) for the bladder and small bowel was lower, while still maintaining minimal D2cc (Gy) for the rectum and sigmoid colon, when the BV was less than 300 cc. For the curved type, the D2cc (Gy) values for OARs were significantly associated with the BV of 100 cc or less. However, the correlation between the bladder and D2cc (Gy) for the E group was not significant, with a p-value of –0.035.
Levine’s test was applied to verify the homogeneity of variance for each group, and indicated significant difference among the groups, with p-value < 0.05 (Table 3). The tilted group demonstrated a significant difference in the average bladder center displacement, with 8.47 mm and 7.11 mm before and after ICBT simulation, respectively (p-value < 0.05), compared with the curved and E groups. In the curved group, the average bladder center displacement was 4.74 mm and 4.40 mm, respectively, and the difference in displacement was significantly less than that in the tilted group (55.9% and 61.9%). In the E group, the average bladder center displacement was 5.11 mm and 5.12 mm, respectively. The center displacement of the bladder measured during ICBT simulation was largest in the tilted group and smallest in the curved group, just as the MRI measurement results obtained before the simulation. The difference in the center displacement of the bladder before and after ICBT simulation was 1 mm, 0.52 mm, and 0.42 mm in the tilted group, curved group, and E group, respectively. As a result of measuring the tandem displacement of the applicator from the mid-sagittal line, the tilted group showed the largest value, with an average of 6.12 mm among the three groups. Table 4 summarizes the measurements of AU and PU lengths in the uterus before and during ICBT simulation. Wilcoxon signed-rank test was performed to evaluate the significant difference in these lengths according to the bladder type and BV. In all bladder types, p-value was 15.48 mm in the E group. The median differences in the PU decreased in all the groups. It was the narrowest in the E group, with an average value of 17.6 mm.
Table 4
Changes in the length of anterior and posterior cervical spaces before and during ICR simulations to evaluate the effect of each bladder type on bladder and small bowel D2cc (Gy)
Discussion
In previous studies, several researchers have reported findings on determining the appropriate BV during intra-cavitary brachytherapy with 3D IGBT. Sun et al. documented a 48% reduction in the median bladder wall absorbed dose, D50 (Gy), when the bladder was full (median volume of 200 cm3) compared with empty bladder. This resulted in decreased acute reactions and long-term complications [20]. However, the maximum bladder dose, D5 (Gy), did not show a significant difference between bladder emptying and filling. In a study of Yamashita et al., the authors observed that the maximal bladder dose (D2cc) increased proportionally with the BV, while the dose to the small bowel (D2cc) decreased. Nevertheless, no significant correlation was found between doses to the rectum, sigmoid-colon, and bladder distension [21]. Both prospective studies used a Fletcher type applicator, and changes were observed in OAR dose as the bladder was filled from an empty state. Despite confirming that BV changes influenced the bladder dose, these studies did not establish the underlying causes for these variations. Moreover, the recent adoption of Vienna ring or Venezia applicators may introduce discrepancies from current practices.
Remarkably, both the bladder and rectal sigmoid colon dose distributions yielded outcomes similar to those of the previous prospective studies, even within retrospective analysis. Sharma et al. showed that a 10% increase in the bladder volume during high-dose-rate intra-cavitary brachytherapy for cervical cancer treatment led to around a 5% rise in radiation dose to the rectum, and a 3% increase to the sigmoid colon [8]. This emphasizes the sensitivity of these organs to changes in bladder volume, and the potential for higher radiation doses with larger bladder volumes. In a retrospective analysis, Ma et al. evaluated data of 166 patients, and examined the incidence and survival rates of cystitis and proctitis based on BV classification, a novel aspect in the research [19]. The optimal survival rate was observed within the range of 100 ml to 150 ml BV, accompanied by a low incidence of proctitis and cystitis. However, BV exceeding 200 ml demonstrated better outcomes than BV within the range of 150 ml to 200 ml. Consequently, determining the most suitable BV proved challenging in their study. Mahantshetty et al. reported a shift in OAR dose due to bladder distension (empty, 50 ml, and 100 ml) using a ring type applicator in 3D-optimized HDR-ICBT [7]. They measured the displacement of D2cc BV in three directions as the bladder expanded. Interestingly, the increase in BV did not significantly impact the movement of D2cc BV. Notably, the focus was on lateral movement of D2cc BV. Although the center of BV showed movement with expansion in anterior-posterior, head-to-caudal, and lateral directions, lateral movement displayed a significantly larger distorted value compared with the other two movements. Instances with a small BV yet high center displacement exhibited a ratio similar to those without such displacement. In summary, lateral movement did not occur with increased BV in specific cases, suggesting the influence of factors beyond BV on this movement. To confirm this, we measured lengths from the applicator’s center to the left and right bladder borders, revealing a greater difference in average lengths for T type bladders. Compared with previous prospective studies, this study confirmed that the pattern, in which bladder distension increases bladder dose (Gy) D2cc and decreases small bowel dose (Gy) D2cc, may vary based on the type of the bladder.
Previous literature present inconsistent findings regarding the appropriate BV, and a consensus on this matter has not yet been achieved [7, 8, 17-19]. In our own clinical experience, we observed discrepancies in radiation dose to OAR even with the same bladder volume. Similarly, Mahantshetty et al. found that bladder expansion have a more significant impact on lateral displacement. These observations served as a significant motivation for our exploration into bladder morphology. Interestingly, prior literature did not address the impact of bladder shape. Therefore, this study aimed to establish a potential correlation between irradiated OAR dose and bladder volume within morphologically classified bladder types, with the goal of determining the appropriate BV. In order to achieve this, the study classified bladder types and categorized volumes using the collected data. It was hypothesized that these factors depend on bladder shape.
The current study meticulously examined bladder morphology among the participants, and identified two distinct bladder types: Type 1: The bladder tilts more to one side; Type 2: The bladder assumes a curved shape on both sides around the applicator. If these two types were not relevant, patients were categorized into third group (the E group). As the data from these three groups did not follow a normal distribution, the significance probability was assessed with Spearman’s rank test, confirming non-parametric correlation. The analysis of relationship between irradiated dose to OAR and each group revealed a significant correlation in the groups (Table 2). Notably, patients in the E group lacked a significant correlation between BV changes and changes in small bowel dose. This could be attributed to the bladder and applicator positions falling outside the range of influence, comparable with those of the tilted or curved groups. In this study, the positional interaction between the uterus and ring type applicator induced by bladder distension, influenced the OAR dose in brachytherapy. Despite an increased BV, the reason behind the inability to reduce the dose to the small bowel in the E group could not be confirmed. Nevertheless, the finding that changes in the bladder volume hold significance for a specific bladder shape emphasizes the importance of considering not only volume but also shape when determining the appropriate BV.
To assess whether bladder shape could be classified using MR images taken before ICBT simulation, several indicators were measured based on images captured before and after simulation. Lengths from the center to outer bladder borders on the left and right sides were measured, and the difference before and after ICBT simulation was evaluated using ANOVA with non-parametric data. The results revealed a significant difference in length, with a p-value of less than 0.01 (Table 3). According to Table 3, the mean difference in the center to right and center to left bladder distances obtained from MR images before and during ICBT simulation was found to be less than 1.3 mm for each bladder type. This suggests that pre-ICBT MR images can be used as criteria for determining bladder types. Remarkably, within the tilted group, the average discrepancy in bladder center displacement was 8.47, significantly greater than that observed in the other groups. The ability to identify the T type bladder using pre-scanned MR images proves to be highly valuable in determining the optimal BV. Furthermore, Wilcoxon signed-rank test that assessed the change before and during intra-cavitary radiotherapy simulation, revealed a significant difference (p-value < 0.01) among the three groups in terms of changes in the front and back lengths of the uterus (Table 4).
The current study has limitations. The evaluation was conducted using retrospective data, which indicated a statistical weakness due to uneven distribution of BV across the three groups. This study applied a treatment protocol that used manual optimization to maintain the irradiated dose to HR-CTV D90% within a certain range. Due to this approach, it was difficult to apply the same evaluation method to every patient. Considering this limitation, we believe that future studies can implement a treatment planning method that maintains consistent optimization. MR images used for dose analysis and based on bladder morphology acquired before and after treatment, included variations due to patient positioning, continuous bladder filling, or the presence/absence of an applicator. These variations could affect the position of the applicator and consequently, the bladder shape. Treatment data utilized in this retrospective study did not undergo monitoring to observe or adjust for these changes. Therefore, factors, such as changes in position of the applicator, or patient positioning were not reflected in the results of analysis, ultimately constituting a limitation of this study. Therefore, the limitations of this study prevent it from being considered sufficient for clinical practice. Nevertheless, from a clinician’s perspective involved in ICBT treatment, the key concern lies in the varying volume of bladder filling based on the bladder type. To ensure more effective clinical application in the future, a prospective study with local control rate, incidence rate of side effects, such as cystitis and proctitis, and development of a predictive model for post-procedure bladder shape, is essential.
Conclusions
This study establishes the substantial impact of bladder distension on OAR doses, primarily attributed to the positional interactions between the uterus and applicator. Notably, our findings emphasize a significant correlation between the increased maximum bladder dose and decreased maximum small bowel dose within the tilted and curved groups. Importantly, within these specific groups, the challenge of achieving predictable results while adhering to the EMBRACE guidelines for maximum small bowel dose by increasing bladder volume, becomes more apparent. Within these groups, we observed a distinct difference in the length of outer bladder border from the midline when comparing pre-ICBT simulation and during ICBT simulation MR images.
In summary, this study underscores the utmost importance of considering the bladder shape when determining the appropriate bladder filling volume, particularly for effectively managing the bladder and small bowel doses. Furthermore, our findings suggest that pre-simulation images can serve as a valuable tool for predicting post-simulation bladder shape, contributing to the optimization of cervical cancer treatment planning.