Introduction
Dendritic cells (DCs) are professional antigen-presenting cells that play a central role in both innate and adaptive immunity [1, 2]. The occurrence and development of various diseases such as allergies, cancer, inflammation, and autoimmune diseases are associated with DCs [3–6]. The surface markers CD86 and CD11c of DCs are expressed during their activation, which contributes to a variety of immune responses such as antigen presentation, T-cell expansion, and others [1, 7, 8]. There is a lot of toll-like receptor 4 (TLR4) on the surface of DCs, and the activation of the TLR4 signalling pathway is beneficial to promote the maturation of DCs [9, 10]. Activation of the TLR4/interleukin-1 receptor-associated kinase 4/nuclear factor kappa-B (IRAK4/ NF-κB) signalling pathway ultimately leads to the release of pro-inflammatory cytokines, which are associated with the pathogenesis of various diseases [11, 12]. Quercetin (QUE), a natural flavonoid, is known for its antioxidant, anti- allergic, anti-cancer, anti-viral, anti-bacterial, and anti-inflammatory properties [13–15]. In this study, we investigated the effect of QUE on the activity and function of DCs via the TLR4/IRAK4/NF-κB signalling pathway. We hope to gain a deeper understanding of the diagnostic and therapeutic targets and potential mechanisms of QUE on DC-related diseases.
Material and methods
Experimental materials
Dendritic cells were the mouse DC 2.4 cell line purchased from ATCC (http://www.atcc.org/). Quercetin (Puxi, China); lipopolysaccharide (LPS) (Sigma-Aldrich, China); TLR4 (Beyotime, China); NF-κB p65 (CST, USA); IRAK4 (Abcam, USA); BCA kit (Thermo, USA); FITC anti-CD86 antibody and PE anti- CD11c antibody (BD Biosciences, USA); Cell Fixation Membrane Breaker (eBioscience, USA); SDS-PAGE electrophoresis system, Western blotting transfer system, ChemiDoc MP multifunctional chemiluminescence imaging system (Bio-Rad, USA); BD FACSCanto II flow cytometer (BD Bioscience USA); Enzyme labeller SpectraMax Paradigm MultiMode Microplate Reader (Molecular Devices, USA).
Experimental methods
Effects of different concentrations of quercetin on dendritic cells at different action times
Dendritic cells were cultured in 96-well plates (5000 cells/well) and incubated for 24 hours at 37°C in an incubator with 5% CO2. Quercetin was dissolved with dimethyl sulfoxide (DMSO) and the concentration of DMSO was controlled to be ≤ 0.1%. Blank groups and normal control (NC) groups were set up separately. Different concentration gradients of QUE (concentration range 0–200 µmol/l) were added into 96-well plates to continue incubation in the incubator, with 3 replicate wells for each concentration. 10 µl of CCK-8 solution was added after 24, 48, and 72 hours, respectively. The optical density (OD) at 450 nm of each group was measured on an enzyme labeller after continuing to incubate for 1 hour in the incubator. The inhibition rate of QUE on DCs was calculated and plotted.
A normal control (NC) group, LPS group, QUE (50 µmol/l) group, and QUE (100 µmol/l) group were set up. Dendritic cells were cultured in 6-well plates (1.5 million cells per well) and incubated for 24 hours at 37°C in an incubator with 5% CO2. Corresponding drugs were added to each group: nothing to the NC group, LPS (1 µg/ml), and QUE (50 µmol/l and 100 µmol/l). Cells were collected after 24 hours of continued incubation, washed 2 times with phosphate buffer saline (PBS), and centrifuged. Binding buffer 500 µl, annexin V 5 µl, and propidium iodide (Pi) 5 µl were added. Then they were incubated for 5 minutes at room temperature away from light, filtered, and assayed on a flow cytometer.
Relative expression of TLR4, IRAK4, and NF-κB p65 proteins
A normal control group, LPS group, QUE group, IRAK4-IN-4 group, LPS+QUE group, and LPS+IRAK4-IN-4 group were set up. Dendritic cells were cultured in 6-well plates (1.5 million cells per well) and incubated for 24 hours at 37°C in an incubator with 5% CO2. Corresponding drugs were added to each group: nothing to the NC group, LPS (1 µg/ml), QUE (100 µmol/l), and IRAK4-IN-4 (1 µg/ml). Cells were collected after 24 hours of continued incubation. The supernatants were collected as the proteins stock solution after lysing with cell lysate, and the protein concentrations were measured by BCA kit. The protein concentration of each group was adjusted to the same and then the samples were loaded at the amount of 30 µg for SDS-PAGE electrophoresis. The voltage was adjusted for electrophoresis. Subsequently, the proteins were transferred to PVDF membranes and closed with tris buffered saline with tween (TBST) containing 5% skimmed milk powder for 1 hour. Membranes were cut, and primary antibodies (glyceraldehyde-3-phosphate dehydrogenase 1 : 2000, TLR4 1 : 2000, NF-κB p65 1 : 1000, IRAK4 1 : 1000) were applied and shaken on a shaker in a refrigerator at 4°C overnight. The membranes were rewarmed at room temperature for 1 hour and washed 3 times with TBST for 10 minutes each time. Then the membranes were incubated with goat anti- rabbit IgG antibody (1 : 5000) for 2 hours and washed again 3 times with TBST for 10 minutes each time. The results were analysed by ImageJ software after development.
Changes in the percentage of CD86 and CD11c on the surface of dendritic cells
A normal control group, LPS group, QUE group, and IRAK4-IN-4 group were established. Dendritic cells were cultured in 6-well plates (1.5 million cells per well) and incubated for 24 hours at 37°C in an incubator with 5% CO2. The corresponding drugs were added and incubated for 24 hours according to the above grouping. Cells were collected and washed twice with PBS. CD86 and CD11c antibodies (1 µl of each) were added and incubated for 30 minutes at 4°C in a refrigerator in the dark (CD86 and CD11c single-stained tubes and a blank control tube should be set separately). After being centrifuged, the supernatant was discarded and washed 2 times with PBS before being assayed on a flow cytometer. Results were analysed with Flowjo software.
The binding site and free energy of quercetin and IRAK4
The target protein IRAK4 protein data bank identity [(PDB ID): 6thx] was obtained by searching the PDB structure database (https://www.rcsb.org/), and it was imported into PyMOL to remove the water molecules as well as the original ligands, and then imported into the AutoDock Tools 1.5.6, hydrogenated, set as receptor, and saved as pdbqt format. The sdf 3D structures of QUE and the above target protein inhibitors (IRAK4-IN-4, CHIR-98014, Selisistat) were obtained from the PubChem database, and minimum energy optimization was performed with an MM2 force field of Chem3D, and the results were saved in mol 2 format. AutoDock Tools1.5.6 hydrogenates small molecules, set them as ligands, detected and set torsional bonds, and finally saved them in pdbqt format. The docking parameters of AutoDock Vina software were set with AutoDock Tools 1.5.6, with a docking count of “num_modes = 20”, a docking box size of the whole protein, and other defaults. The minimum binding energy conformation was exported as a pdb file, and the ligand-protein complex was generated with PyMOL, and then imported into Discovery studio software, hydrogenated to show the interactions, and finally visualized.
Statistical analysis
Data were statistically analysed and graphed using GraphPad Prism 8.0 software. Measurement data were expressed as (x̄ ±s), Student’s t-test was used for two-way comparison, and one-way ANOVA was used for the comparison between multiple groups; p < 0.05 was considered statistically significant.
Results
Quercetin inhibited the activity and function of dendritic cells in a time-dose-dependent manner
We observed the effect of different concentrations of QUE on DCs at different periods. The results of our CCK-8 assays showed that after DCs were treated with QUE at concentration gradients of 0 µmol/l, 12.5 µmol/l, 25 µmol/l, 50 µmol/l, 100 µmol/l, and 200 µmol/l for 24, 48, and 72 hours, the inhibition rate of QUE on DCs was gradually enhanced with the increase of QUE dose and action time. The inhibition rate gradually reached saturation with the increase of QUE concentration and action time. The results for the survival rate of DCs were the exact opposite of those for the inhibition rate of DCs. When the QUE concentration was 100 µmol/l and 200 µmol/l, the survival rate and inhibition rate of DCs for 48 and 72 hours were statistically significant compared with that for 24 hours. The results of the apoptosis assays showed that the rate of living DCs (annexin V-/Pi-) decreased with increasing QUE concentration when different concentrations of QUE were applied to DCs. The higher the QUE concentration, the fewer living DCs there were. At different action times (24, 48, and 72 hours), QUE began to have significant effects on living DCs when the concentration of QUE was 100 µmol/l, and the difference was statistically significant (Fig. 1). This also indicates that apoptosis of DCs can be significantly induced when the QUE concentration is 100 µmol/l. Therefore, we think that QUE plays a role by affecting the activity and function of DCs. We used a QUE concentration of 100 µmol/l and action time of 24 hours to conduct follow-up experiments.
Fig. 1
The effect of quercetin on dendritic cells (DCs) (n = 3 per group, error bars are mean ±SD). With the prolongation of the action time and the increase of QUE concentration, the inhibition rate of QUE on DCs gradually increased. When the concentration of QUE was 100 μmol/l and the action time was 24 hours, QUE can significantly affect the activity and function of DCs
DCs – dendritic cells, LPS – lipopolysaccharide, NC – normal control, PI – propidium iodide, QUE – quercetin
* p < 0.05
** p < 0.01
*** p < 0.01
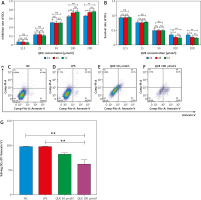
Quercetin inhibited the relative expression of TLR4, IRAK4, and NF-κB p65 proteins induced by lipopolysaccharide
We detected the relative expression of TLR4, IRAK4, and NF-κB p65 proteins by Western blotting. The results showed that the relative expression of TLR4, IRAK4, and NF-κB p65 proteins in the LPS group was significantly higher than that in the NC group, and the relative expression of these proteins decreased after treatment with QUE and IRAK4-IN-4 compared with the LPS group. In the LPS+QUE group and LPS+IRAK4-IN-4 group, the relative expression levels of the above proteins were between the LPS group and QUE and IRAK4-IN-4 group. We also observed that the effect of QUE on the above proteins was comparable to that of IRAK4-IN-4. This suggests that QUE inhibits the expression of TLR4, IRAK4, and NF-κB p65 proteins induced by LPS, and its inhibition effect may be related to IRAK4 (Fig. 2).
Fig. 2
Effect of quercetin and IRAK4-IN-4 on TLR4, IRAK4, and NF-κB p65 proteins (n = 3 per group, error bars are mean ±SEM). Relative expression of TLR4, IRAK4, and NF-κB p65 proteins (A). Statistical results of relative expression of TLR4, IRAK4, and NF-κB p65 proteins (B–D). Lipopolysaccharide group compared with NC group. LPS group compared with the QUE and IRAK4-IN-4 group
** p < 0.01
### p < 0.001
####p < 0.0001 C
IRAK4 – interleukin-C receptor-associated kinase 4, LPS – lipopolysaccharide, NC – normal control, QUE – quercetin
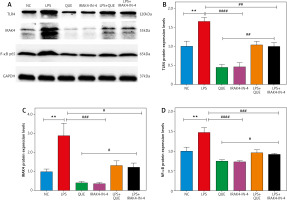
Quercetin inhibited the expression of CD86 and CD11c on the surface of dendritic cells
We detected the ratios of CD86- and CD11c-positive cells on the surface of DCs by flow cytometry, respectively. Our results showed that the positive cell ratios of CD86 and CD11c were significantly increased in the LPS group and decreased after treatment with QUE and IRAK4-IN-4. This indicated that QUE inhibited the expression of CD86 and CD11c on the surface of DCs induced by LPS, and its inhibition rate was comparable to that of IRAK4-IN-4 (Fig. 3).
Fig. 3
Effect of quercetin (QUE) on the ratio of CD86- and CD11cpositive cells on the dendritic cells surface (n = 3 per group, error are the mean ±SEM). The ratio of CD86- and CD11c-positive cells among the various groups, separately (A–D, F–I). Statistical graph of the ratio of CD86 and CD11c positive cells, separately (E, J). Lipopolysaccharide group compared with the normal control group. Lipopolysaccharide group compared with the QUE and IRAK4-IN-4 group
*** p < 0.001
**** p < 0.0001
# p < 0.05
#### p < 0.0001
IRAK4 – interleukin-1 receptor-associated kinase 4, LPS – lipopolysaccharide,
NC – normal control, QUE – quercetin

The binding sites of quercetin and IRAK4 were stable, with the minimum binding energies comparable to that of IRAK4-IN-4
Our molecular docking results showed that QUE and IRAK4 could interact with each other, and they had good binding sites with a minimum binding energy of 9.6 kcal/mol, which was comparable to that of IRAK4-IN-4 with a minimum binding energy of 9.7 kcal/mol. The lower the binding energy, the more stable the bond between them. This suggests that IRAK4 may be a target of QUE (Fig. 4).
Fig. 4
Molecular docking simulation diagram of quercetin (QUE) with interleukin-1 receptor-associated kinase 4. Superposition diagram of molecular docking interactions (A). Detailed view of molecular docking pocket interactions (B). Diagram of the 2D virtual model of molecular docking (C). We can see that the QUE binding site was located in the action pocket formed by amino acid sites such as Tyr262, Tyr263, and Met256. The 2D virtual map shows that QUE forms a Pi-Pi bond with Tyr262 and forms 4 hydrogen bonds with Lys213, Met192, and Tyr264
IRAK4 – interleukin-1 receptor-associated kinase 4, PDB ID – protein date bank identity, Pi – Π, QUE – quercetin
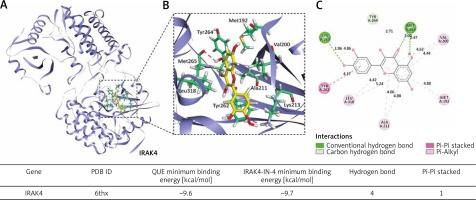
Discussion
Dendritic cells are a class of bone marrow-derived cells arising from lympho-myeloid haematopoiesis that can be categorized into conventional DCs, plasmacytoid DCs, monocyte-derived DCs, and Langerhans cells [2, 16]. The innate and adaptive immune systems are connected by DCs, which are also essential for initiating and controlling immune responses [16]. Generally, DCs are resting cells, and once activated they increase the expression levels of surface MHC-peptide complexes and costimulatory molecules, which allows them to efficiently activate T-cells [1]. The immune response induced by DCs is closely related to T-helper type 1 (Th1)/T-helper type 2 (Th2), regulatory T-cells (Tregs)/T-helper type 17 (Th17) [17]. The imbalance of Th1/Th2 and Tregs/Th17 is related to various diseases such as allergies, inflammation, and cancer [18–21]. However, DCs are both tolerogenic and immunogenic, and tolerogenic DCs induce immune tolerance by stimulating Tregs or producing cytokines that inhibit T-cell activation [22]. This will help to treat diseases related to DCs.
Quercetin is a natural flavonoid compound found in a variety of fruits and vegetables, with a wide range of biological benefits [13, 23]. It is almost regarded as a panacea. After applying QUE to DCs, we found that it had a significant inhibitory effect on DCs, suggesting that the therapeutic effect of QUE may be related to DCs. Our experimental results showed that the inhibitory effect of QUE on DCs increased with the increase of QUE concentration. When we continuously increased the concentration of QUE, its inhibitory effect tended to be stable. According to the results of our CCK-8 and apoptosis assays when the concentration of QUE was 100 µmol/l and the action time was 24 hours, the number of living DCs was significantly reduced, which indicates that QUE causes DC apoptosis in this case, thus affecting the activity and function of DCs, which in turn contributes to the treatment of diseases associated with DCs. This is consistent with previous reports that QUE can significantly induce DC apoptosis when the concentration is 100 µmol/l [15]. The concentration and timing of QUE action on DCs will contribute to subsequent experimental studies or drug development.
So, what mechanism does QUE enact on DCs? We continued the Western blotting experiments to explore whether QUE inhibits the activity and function of DCs via the TLR4/IRAK4/NF-κB signalling pathway by observing the effects of QUE on related proteins. Our experimental results showed that QUE inhibited the relative expression of TLR4, IRAK4, and NF-κB p65 proteins, and its inhibitory effect was comparable to that of IRAK4 inhibitor IRAK4-IN-4 (Fig. 2). This shows that QUE can inhibit the expression of related proteins in the TLR4/IRAK4/ NF-κB signalling pathway induced by LPS. We speculate that the inhibitory effect of QUE on the activity and function of DCs is associated with this signalling pathway.
There are many TLR4 receptors on the surface of DCs, and their activation is beneficial to regulate the antigen presentation of DCs [9]. Lipopolysaccharide transduces signals mainly via TLR4 [24]. With the help of LPS-binding protein and CD14, TLR4 binding to LPS prompts the activation of the TLR4/IRAK4/NF-κB signalling pathway, which ultimately results in the release of a variety of cytokines, such as TNF-α, IL-1β, IL-2, IL-6, and COX-2 [25–27]. These cytokines will be involved in the development and progression of multiple diseases.
Which target does QUE specifically act on in the TLR4/IRAK4/NF-κB signalling pathway? When DCs are acti- vated, their surface markers CD86 and CD11c are upregulated, which may be related to TLR4 signalling pathway [28, 29]. We then performed flow cytometry experiments to compare IRAK4 inhibitor IRAK4-IN-4 and QUE, and we found that QUE and IRAK4-IN-4 had comparable inhibition rates to the marker molecules CD86 and CD11c on the surface of DCs (Fig. 3). Our flow cytometry results coincide with the results of the Western blotting experiments, which further increases the possibility that QUE acts on the target of IRAK4 in the TLR4/IRAK4/NF-κB signalling pathway.
It is theoretically possible to predict the binding modalities and affinities of small molecule ligands by using molecular docking to examine the interaction and recognition between protein receptors and ligands [30]. The level of binding energy usually reflects the activity between the compounds and the protein targets, i.e. lower is more stable [31]. To further verify our speculations, we performed the molecular docking binding sites of QUE and IRAK4 and calculated their binding free energies. We found that the binding sites of QUE and IRAK4 were stable and that QUE had comparable binding free energies to the IRAK4 inhibitor IRAK4-IN-4 (Fig. 4). This further adds to the supporting evidence that QUE acts on IRAK4 targets in the TLR4/IRAK4/NF-κB signalling pathway.
Conclusions
We concluded that QUE may inhibit the activity and function of DCs via the TLR4/IRAK4/NF-κB signalling pathway, and IRAK4 may be its target. However, QUE may affect the activity and function of DCs through a variety of pathways, and its targets are not only limited to IRAK4. Because there may be more targets, we need to conduct more experiments to understand the effect of QUE on the acti- vity and function of DCs in a comprehensive manner. This will help treat diseases associated with DCs through multiple pathways. In addition, whether different concentrations of QUE (especially high concentrations) have toxicity and side effects while treating diseases associated with DCs needs to be further explored.