Introduction
In the last decade, significant progress has been made in understanding the role of nitric oxide (NO) in the skin. It has been shown that NO, being secreted by various skin cells, at physiological concentrations plays a role in the maintenance of cellular homeostasis, regulates blood flow in the skin microvasculature, and is an active component of the skin immune system. On the other hand, excessive and prolonged production of NO in the skin induces local adverse reactions that can lead to various dermatological diseases, from pigmentary disorders to neoplastic lesions. Convincing evidence exists that NO is a mediator of the diverse activity of ultraviolet radiation (UVR) in the body [1–3]. A strong impulse to increase the endogenous NO level in the skin is stress caused by skin wounding, exposure to UV radiation, or infection. In response to such insults, the immune-competent skin cells, such as dendritic and mast cells, keratinocytes, fibroblasts, and melanocytes, start to enhance NO production and secretion via activation of inducible nitric oxide synthase (iNOS). In this respect, melanin-producing melanocytes, the main constituents of the skin pigmentation system, can be regarded as local “stress sensors” in the epidermis [1, 2, 4]. Numerous factors have been shown to activate iNOS expression in melanocytes, including bacterial lipopolysaccharide (LPS), proinflammatory mediators tumor necrosis factor α (TNF-α), and interferon-γ (IFN-γ), and UV radiation [5–9]. Epidermal melanocytes are also capable of releasing NO spontaneously, due to the constitutive expression of the endothelial and neuronal isoforms of NOS [10, 11]. Furthermore, UVA and short-wave visible radiation can cause enzyme-independent production of large amounts of NO in the skin, thereby inducing changes in melanocyte physiology [3]. Studies on in vitro cell models strongly suggest that NO present in the surrounding cellular environment can affect melanocyte physiology in a paracrine and/or autocrine manner [12]. Importantly, no such effects were observed at low NO concentrations or for short-term exposure, whereas high NO concentrations were cytotoxic and decreased cell viability [13]. It has been reported that NO induces detachment of melanocytes from the extracellular matrix, which presumably contributes to pigment cell loss in vitiligo or melanoma metastasis [14–16]. Also, clinical studies indicate that disruption of normal NO production in the skin can lead to severe modifications of melanocyte function and even cell destruction, thus being the cause of pigmentary disorders or neoplastic transformation [12].
There is some evidence that NO plays a role in the modulation of melanogenesis [6, 7, 17–21]. Human epidermal melanocytes produce 2 chemically distinct types of melanin pigment eumelanin and pheomelanin [22–25]. The cell melanin content and composition (i.e. the proportion of its pheomelanin component) depends on the activity of key melanogenic enzymes inside melanocyte, such as tyrosinase (TYR), tyrosinase-related protein 1 (TYRP1), and dopachrome tautomerase (DCT), and on the cellular availability of melanin precursors, including the sulphur-containing compounds that are essential for pheomelanin formation [26, 27]. NO released in the skin from cells surrounding melanocytes (and probably from melanocytes themselves) when exposed to UV radiation has been shown to stimulate melanin synthesis and alter the ratio of eumelanin to pheomelanin in the skin by enhancing eumelanogenesis [6, 7, 17–19]. To date, little is known about whether constitutive cell pigmentation influences the sensitivity of melanocytes to NO. Ivanova et al. [15] reported that more pigmented normal human melanocytes and vitiligous melanocytes were more sensitive to NO-induced detachment from the extracellular matrix than less pigmented melanocytes. We have previously found substantial differences in the LPS-stimulated expression of numerous genes encoding inflammatory cytokines and chemokines between human epidermal melanocytes with various pigmentary phenotypes [28].
Aim
The present study was designed to compare the effect of NO on melanin production and the expression of melanogenesis-related genes (TYR and DCT) in normal human melanocytes derived from lightly and darkly pigmented skin and cultured in the presence of nitric oxide complex with spermine (SPER/NO).
Material and methods
Reagents and compounds
Spermine/nitric oxide complex (N-[4-[1-(3-Aminopropyl)-2-hydroxy-2-nitrosohydrazino]butyl]-1,3-propanediamine, SPER/NO), 3,4-dihydroxyphenylalanine (L-DOPA), L-cysteine, 3-methyl-2-benzothiazolinohydrazone (MBTH), N, N’-dimethylformamide (DMF), Dulbecco’s Phosphate Buffered Saline (D-PBS), and Trypan Blue solution (0,4%) were purchased from Sigma-Aldrich Inc. (St. Louis, MO, USA). Growth medium M-254, Human Melanocyte Growth Supplement (HMGS), antibiotic/antimycotic solution of gentamicin and amphotericin B, and Trypsin/EDTA solution were obtained from Cascade Biologics/GIBCO Life Technologies (Carlsbad, CA, USA).
Cell culture
The commercially available human epidermal melanocytes of neonatal origin derived from a lightly pigmented (HEMn-LP, lot# 200709-589) and a darkly pigmented donor (HEMn-DP, lot# 1107461) (Cascade Biologics/GIBCO Life Technologies, Carlsbad, CA, USA) were used. The cells were cultured in M-254 basal medium supplemented with HMGS, gentamicin (10 μg/ml), and amphotericin B (0.25 μg/ml) at 37°C in 5% CO2. All experiments were performed using cells in passages 3–5.
Estimation of cell viability
The parameters used for evaluating melanocyte viability were LDH leakage and 5-bromo-2'-deoxyuridine (BrdU) incorporation. The cells were incubated for 24 h with culture media containing 50 or 100 μM SPER/NO. The cytotoxicity of the NO donor was evaluated using CytoTox 96® Non-Radioactive Cytotoxicity Assay (Promega, Madison, WI, USA), which measures released lactate dehydrogenase (LDH) in cell culture supernatants. Cell proliferation was assessed based on the measurement of BrdU incorporation during DNA synthesis using Cell Proliferation ELISA BrdU (Roche, Basel, Switzerland) according to the manufacturer’s protocol.
Measurement of melanin content in cultured melanocytes
Single and repeated-dose studies were conducted to investigate the effect of SPER/NO on melanin content. For this purpose, HEMn-LP and HEMn-DP were exposed to the NO donor (50 and 100 μM) for 3 or 6 days. In the 3-day experiment, the cultured cells were supplemented with SPER/NO only once, whereas in the 6-day experiment, a fresh dose of the NO donor was added every other day during culture medium replacement. After the incubation period, the cells were detached by trypsinization, counted using a haemocytometer, and washed with D-PBS. Then, 40,000 cells were lysed by the freeze/thaw cycle, and the remaining pellets were solubilized in 1 ml 1 M NaOH (10-hour incubation at ambient temperature, then heating at 50°C for 1 h). After centrifugation, the absorbance of the obtained supernatants (250 μl) was measured in a 96-well plate at 450 nm with a microplate reader (Triad LT Multimode Detector, Dynex Technologies, Chantilly, VA). Melanin content was calculated using a standard curve of synthetic DOPA-melanin and expressed as pg per cell.
Melanin analysis by pyrolysis-gas chromatography/tandem mass spectrometry (Py-GC/MS/MS)
The frozen cell pellets of HEMn-LP and HEMn-DP cultured with or without 50 μM SPER/NO for 6 days were used for melanin isolation according to the previously described protocol [29]. Briefly, the cells were thawed and lysed with 1% Triton X-100. After centrifugation, the remaining pellets were incubated with sodium dodecyl sulphate and proteinase K. The insoluble melanin was collected by centrifugation, extensively washed with organic solvents, and dried to a constant weight at 37°C. Then the pheomelanin contents of the isolated pigments were quantitated using the described earlier method based on GC/MS/MS analysis of their marker pyrolysis products [30]. The instrumentation used and conditions for Py-GC/MS/MS analysis are described in the Supplementary material.
Measurement of tyrosinase activity
The DOPA oxidase activity of tyrosinase in melanocytes was determined using the dopachrome method with slight modification. Briefly, lightly and heavily pigmented melanocytes were treated with SPER/NO for 24 h. After the treatment, the cells were detached with trypsin–EDTA, quantitated using a haemocytometer, washed with D-PBS, and lysed by the freeze/thaw cycle followed by incubation with 1% Triton X in phosphate buffer (pH 6.8) at ambient temperature for 30 min. The lysate was then centrifuged at 15,000 g for 10 min. An aliquot of the resulting cell extract corresponding to 40,000 cells was transferred directly to a quartz cuvette, and a solution of L-DOPA (1 mM) in 50 mM phosphate buffer (pH 6.8) was added to a final volume of 1 ml. Dopachrome formation was monitored by measuring the increasing absorbance at 475 nm (UV-VIS spectrophotometer Jasco V-630) every 1 s. Tyrosinase activity was then determined using the initial slope of the recorded kinetic curve (reaction in initial 30 s) and the molar absorption coefficient of dopachrome.
Tyrosinase activity was also determined using the MBTH assay based on Winder and Harris with slight modification [31, 32]. The cells were cultured and prepared in the same way as in the case of the dopachrome method. The reaction mixture (total volume of 1 ml) consisted of L-DOPA (2 mM), MBTH (4 mM), DMF (2%), and an aliquot of the resulting cell extract corresponding to 40,000 cells, in 50 mM phosphate buffer (pH 6.8). The formation of MBTH-dopaquinone adduct was monitored by measuring the increasing absorbance at 505 nm every 1 s. Tyrosinase activity was calculated based on the initial (up to 30 s) slope of the kinetic curve and the molar absorption coefficient of the resulting product.
Quantitative determination of tyrosinase
A TYR (Human) ELISA Kit (Biomatik, Cambridge, ON, Canada) was used to determine tyrosinase concentration. The cells were incubated for 24 h with culture media containing SPER/NO. Next, the cells were washed and resuspended in D-PBS, lysed by repeated freeze-thaw cycles, and centrifuged (1000 g; 4°C; 20 min). The supernatants were used for the determination of tyrosinase according to the manufacturer’s protocol. The absorbance at 450 nm was measured using a microplate reader (Triad LT Multimode Detector, Dynex Technologies, Chantilly, VA). The concentration of tyrosinase was determined based on the prepared standard curve.
RNA isolation and real-time PCR analysis
Total RNA was extracted from homogenates of cells in TRI Reagent® using the Direct-zol™ RNA MiniPrep Kit (Zymo Research, Irvine, CA, USA) according to the manufacturer’s protocol. To remove possible contamination of DNA, samples were treated with DNase I solution. To remove PCR inhibitors, such as melanin, an additional sample purification procedure was performed using the OneStep™ PCR Inhibitor Removal Kit (Zymo Research, Irvine, CA, USA). The amount of isolated RNA was checked using the MaestroNano spectrophotometer (Maestrogen, Hsinchu, Taiwan). First-Strand cDNA synthesis was performed with 1 μg of total RNA, which was transcribed into cDNA using the iScript™ Reverse Transcription Supermix (Bio-Rad Laboratories, Hercules, CA, USA) with oligo (dT) and random primers according to the manufacturer’s protocol. An RT program was performed in a T100 thermal cycler (Bio-Rad Laboratories, Hercules, CA, USA) applying the following thermal profile: 5-minute incubation at 25°C, 20-minute RT at 46°C, and 1-minute RT inactivation at 95°C. The expression of mRNA for TYR, DCT, and GAPDH was determined using the CFX Connect Real-Time PCR Detection System and the SsoAdvanced™ Universal SYBR®Green Supermix (Bio-Rad Laboratories, Hercules, CA, USA). Each reaction contained 10 μl SsoAdvanced™ Universal SYBR®Green Supermix (2×), 1 μl PrimePCR SYBR®Green Assay (20×) for TYR (qHsaCID0014521), DCT (qHsaCID0017860), GAPDH (qHsaCED0038674), 0.5 μl template cDNA, and 8.5 μl nuclease-free water. The reaction conditions were as follows: denaturation at 95°C for 30 s, followed by 40 cycles of 95°C for 5 s and 60°C for 30 s. The specificity of the PCR products was confirmed by melting curves. Each sample was analysed in triplicate, and GAPDH was used as a reference gene. The differences in transcriptional activity of the target genes between treatment and control samples were calculated using the 2-ΔΔCt method.
Results
Cell viability, melanin content, and composition
Within the donor concentration range used, NO released from SPER/NO did not affect the cell proliferation and was not toxic to the treated melanocytes, as shown by the results of the BrdU ELISA and the LDH assay (Table 1).
Table 1
SPER/NO cytotoxicity and the effect of the NO donor on cell proliferation, and melanin content in human epidermal melanocytes in culture (data represent the mean ± SEM)
SPER/NOM [μM] | HEMn-LP | HEMn-DP | ||||||
---|---|---|---|---|---|---|---|---|
Cytotoxicitya | Cell proliferationa | Melanin contentb | Cytotoxicitya | Cell proliferationa | Melanin contentb | |||
3 daysc | 6 daysd | 3 daysc | 6 daysd | |||||
0 | 100 ±12 | 100 ±9 | 28.47 ±2.02 | 32.92 ±1.42* | 100 ±16 | 100 ±10 | 49.99 ±0.96# | 75.14 ±5.43*# |
50 | 97 ±22 | 101 ±7 | 29.33 ±1.90 | 32.20 ±0.64 | 96 ±15 | 97 ±17 | 51.91 ±1.54# | 73.16 ±6.50*# |
100 | 104 ±9 | 111 ±5 | 29.62 ±2.42 | – | 95 ±13 | 102 ±11 | 52.24 ±5.93# | – |
Constitutively, HEMn-DP was found to contain almost twice as much melanin as HEMn-LP. Furthermore, although the pigment levels increased with the cultivation time in both cell types, the efficiency of melanin synthesis was markedly higher in HEM-DP (after 6 days, the ratio of the melanin content of DP cells versus that of LP cells increased from 1.8 to 2.3). The exposure of cells to SPER/NO (50 and 100 μM) did not alter their total melanin content.
Py-GC/MS/MS analysis revealed that melanin produced by the melanocytes growing in the presence of SPER/NO was qualitatively different from the pigment synthesized in the control cells. The relative abundance of sulphur-containing pyrolysis products characteristic of pheomelanin structural units (the pheomelanin markers, P), especially thiazole (P1) and hydroxybenzothiazole (P2), was markedly increased in the pyrolysate of melanin isolated after the cell exposure to the NO donor, while the abundance of the characteristic pyrolysis products devoid of sulphur (NS) remained relatively stable (Figure 1). Such pyrolysis profiles suggest that human epidermal melanocytes, especially those from darkly pigmented skin, produce more pheomelanin when cultured with the NO donor. Indeed, as shown in Figure 2, the pheomelanin content calculated for SPER/NO-treated HEMn-DP was substantially higher as compared to the control cells (45.4% vs. 11.9%). For HEMn-LP cultured under the same conditions, the proportion of pheomelanin was also increased, but to a much lesser extent (5.5% vs. 4.6% calculated for the control).
Figure 1
TIC MRM chromatogram of the pheomelanin markers (P) and characteristic non-sulphur-containing compounds (NS) formed during pyrolysis of melanin isolated from the heavily pigmented melanocytes (HEMn-DP). The cells were cultured without (panels a1 and a2) or in the presence of 50 μM SPER/NO (panels b1 and b2) for 6 days. Peak designation: P1: thiazole, P2: 4-hydroxybenzothiazole, P3–P5: 2,3-dihydro-5H-1,4-benzothiazin-5-one and its isomers, P6 and P7: 7-methyl-2,3-dihydro-5H-1,4-benzothiazin-5-one and its isomer, P8 and P9: 4-hydroxy−6-ethylbenzothiazole and its isomer, P10: 7-methyl-5H-1,4-benzothiazin-5-one, P11 and P12: 7-ethyl-2,3-dihydro-5H-1,4-benzothiazin-5-one and its isomer, NS1: toluene, NS2: styrene, NS3: phenol, NS4: methylphenol, NS5: 1,2-benzenediol, NS6: indole, NS7: methylindole
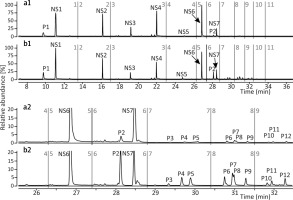
Figure 2
The effect of NO on the pheomelanin content of lightly and heavily pigmented melanocytes (HEMn-LP and HEMn-DP, respectively) cultured for 6 days with and without the addition of 50 μM SPER/NO. Melanin was isolated from the cells and analysed by pyrolysis coupled with gas chromatography and tandem mass spectrometry (Py-GC/MS/MS). The pheomelanin content (%) was calculated from the ratio of the total peak area of all pheomelanin markers and the total peak area of all non-sulphur-containing pyrolysis products, based on a standard curve obtained for synthetic melanins with known percentages of incorporated pheomelanin
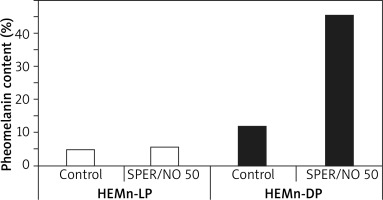
Tyrosinase activity and concentration
As shown in Table 2, constitutive tyrosinase activity in HEMn-DP was approximately 2-fold higher than in HEMn-LP. Neither tyrosinase activity nor the tyrosinase level was changed after 24-hour incubation of the cells with SPER/NO, irrespective of the degree of melanocyte pigmentation and the NO donor concentration used.
Table 2
Tyrosinase activity and concentration in HEMn-LP and HEMn-DP cultured with the addition of SPER/NO (data represent the mean ± SEM); #p < 0.05 between HEMn-LP and HEMn-DP
TYR and DCT gene expression
RT-qPCR results showed that under SPER/NO-free conditions, expression of both TYR and DCT was significantly higher (about 1.5 and 5 times, respectively) in HEMn-DP than in their lightly pigmented counterparts (data not shown). In both HEMn cell types, the constitutive level of TYR and DCT mRNA changed following the exposure to SPER/NO, regardless of the concentration of the NO donor. Moreover, the effect of SPER/NO treatment depended on the cell pigmentation phenotype. In HEMn-LP incubated with SPER/NO (50 and 100 μM) for 12 h, the expression of TYR was found to be upregulated, whereas in HEMn-DP it was slightly downregulated (Figure 3). Under the same conditions, the expression of DCT was slightly increased in HEMn-LP and decreased in HEMn-DP (Figure 4).
Figure 3
Expression levels of TYR in melanocytes treated with SPER/NO (μM). Human epidermal melanocytes from neonatal foreskin (HEMn) were treated with or without SPER/NO for 12 h. Total RNA was isolated and relative TYR expression was measured by RT-qPCR. Fold changes were calculated relative to the untreated control cultures (control value was taken as 1). Data represent the mean ± SEM (n = 3)
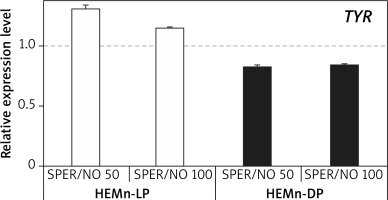
Figure 4
Expression levels of DCT in melanocytes treated with SPER/NO (μM). HEMn were incubated for 12 h with or without SPER/NO. Total RNA was extracted, and relative DCT expression was measured by RT-qPCR. Fold changes were calculated relative to the untreated control cultures (control value was taken as 1). Data represent the mean ± SEM (n = 3)
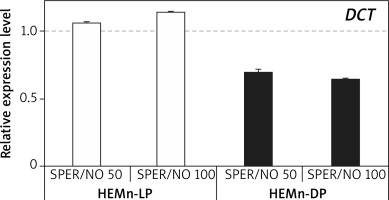
Discussion
We have found that under the experimental conditions used, NO released from SPER/NO did not affect either the total melanin content or TYR activity and concentration in cultured human epidermal melanocytes derived from lightly and darkly pigmented skin. However, the effect of NO on the relative proportion of cellular pheomelanin as well as the expression of the genes encoding TYR and DCT was found to be closely related to the degree of melanocyte constitutive pigmentation.
Our results on melanin content and TYR activity in NO-treated melanocytes in culture are contrary to previous reports. However, another class of NO donor was used in those studies, namely S-nitroso-N-acetylpenicillamine (SNAP). Unlike SPER/NO and other NONOates, the NO donors belonging to the S-nitrosothiol family are not only capable of releasing NO outside the cultured cells but also can act in the cytosol by directly activating guanylate cyclase and thereby the cGMP-dependent protein kinase pathway [33, 34]. The latter mechanism could be responsible for the enhanced melanin synthesis in the cells exposed to SNAP [35].
We have found that melanocytes from darkly pigmented skin cultured with SPER/NO produced far more pheomelanin than the control cells. For melanocytes from lightly pigmented skin, the proportion of pheomelanin produced under the same conditions was only slightly increased compared to the control. The enhanced pheomelanin production in HEMn-LP cultured with the NO-donor (SNAP) was reported earlier by Lasalle et al. [19].
TYR, the key regulatory enzyme involved in melanin biosynthesis, is regarded as a major factor responsible for both the total amount of the pigment produced by epidermal melanocytes and the cell eumelanin/pheomelanin ratio. High activity and availability of TYR are known drivers of eumelanogenesis, while the opposite conditions can shift melanin synthesis towards pheomelanin [36]. Surprisingly, we found no changes in either TYR activity or concentration between melanocytes cultured with and without the NO donor, regardless of the constitutive pigmentation of the cells. However, clear differences were found in the expression levels of the gene encoding TYR before and after the cell exposure to NO, with the observed effect being strictly dependent on the pigmentation phenotype of the melanocytes: TYR expression was increased in HEMn-LP and decreased in HEMn-DP. The change in TYR transcriptional activity was too small, however, to be the sole factor responsible for the very large increase in the pheomelanin content of highly pigmented melanocytes. Therefore, we turned our attention to the gene encoding DCT, another important enzyme that regulates melanogenesis in mammals. DCT isomerizes melanogenic intermediate dopachrome to 5,6-dihydroxyindole-2-carboxylic acid, catalysing one of the final steps leading to eumelanin formation [36]. Guyonneau et al. [37] observed a diluted coat colour phenotype in DCT knockout mice. Furthermore, Costin et al. [38] reported that slaty and slaty light mutations in the DCT-encoding gene dramatically decreased the eumelanin formation and significantly increased the production of pheomelanin by cultured mutant melanocytes derived from the skin of newborn congenic C57BL/6J non-agouti black mice. That observation led the authors to the intriguing speculation that DCT plays a role in the melanocyte switching over to pheomelanin production. In our study, the transcriptional activity of DCT was slightly increased in HEMn-LP and markedly decreased in HEMn-DP after the exposure to SPER/NO. This suggests that NO-induced DCT downregulation could be crucial for the enhancement of pheomelanogenesis in melanocytes from darkly pigmented skin. However, this was certainly not the only causing factor, as indicated by the slightly increased production of pheomelanin in lightly pigmented cells after the exposure to NO, despite clear upregulation of both eumelanogenic genes.
We believe that the observations made in the present work may have important clinical relevance. Previous studies have shown that low levels of NO in the skin microenvironment are essential to maintain epidermal permeability barrier homeostasis [39–42]. Excessive NO can compromise this barrier, which could explain the pathogenic role of NO in inflammatory skin disorders [7, 43]. Darkly pigmented skin was found to display a superior epidermal permeability barrier in comparison with lightly pigmented skin [44–46]. Still, considering several epidemiologic studies, there is a paradoxically greater propensity to develop inflammatory dermatoses in darkly pigmented people, and these disorders tend to be more severe in such individuals [47–52]. It seems likely that pheomelanin, whose content in dark skin may be significantly increased due to the local overproduction of NO, may be another factor inducing or modulating inflammatory dermatoses. Furthermore, it is well known that local chronic inflammation predisposes the skin to carcinogenesis. In this context, it is important to note that pheomelanin is not only structurally different from eumelanin, but its role in cell physiology and pathology is also different. Eumelanin, which is composed of indole-type structural subunits, protects skin cells against UV-induced damage, acting as a sunscreen, antioxidant, and free-radical scavenger, whereas pheomelanin, consisting of benzothiazine/benzothiazole units, can act as an endogenous photosensitizer that generates reactive oxygen species after UV exposure, and it has UV-independent pro-oxidative activity [36]. Melanogenesis itself, especially pheomelanin synthesis, generates reactive oxygen species, including superoxide anion radical, which reacts rapidly with NO to form peroxynitrite. Peroxynitrite can oxidize melanin to carbonyls in an excited triplet state that has an energy equivalent to UV photons [53, 54]. Melanin chemiexcitation has been proposed as one of the mechanisms underlying melanoma development via so-called “dark” cyclobutane pyrimidine dimers (CPD) – the highly mutagenic DNA photoproducts that can be formed in the dark, long after the skin’s exposure to UV irradiation. It was found that pheomelanin was a more potent generator of “dark” CPD than eumelanin [54, 55]. Very recently, a physiological linkage between melanin synthesis, NOS and NADPH oxidase (NOX) activity, and initiation of melanoma from non-cancerous lesions was proposed by Premi et al. [54]. According to their hypothesis, the NOS and NOX activity switches eumelanogenesis to pheomelanogenesis and potentially triggers melanoma from dysplastic nevi by upregulating cellular pheomelanin content. We suspect that NO-induced enhancement of skin pheomelanin content could be also responsible for melanoma de novo formation based on local chronic inflammation, especially in deeply pigmented people.
Conclusions
The current study has provided further evidence that the degree of constitutive pigmentation is an important modulatory factor for melanocyte response to various inflammatory mediators, including NO. We have shown that NO can promote pheomelanogenesis in human epidermal melanocytes and that the cell response in this respect is associated with their pigmentation phenotype. During the exposure to NO, heavily pigmented melanocytes derived from dark skin start to produce much more pheomelanin than their lightly pigmented counterparts. This can be related to the downregulation of key eumelanogenic genes, especially DCT, in HEMn-DP but not HEMn-LP. Further research on lightly and heavily pigmented melanocytes from multiple donors (both neonatal and adult) is needed to fully establish the mechanism by which the cells of various pigmentation phenotypes enhance their pheomelanin content after exposure to NO.