Introduction
Hepatocellular carcinoma (HCC) is the most prevalent primary liver cancer and the second leading cause of cancer-related deaths globally [1]. The annual incidence of HCC in Egypt increased significantly from 4.0% in 1993 to 7.2% in 2003. The burden of HCC incidence in Egypt has doubled in the last 10 years [2]. Chronic viral hepatitis, alcohol misuse, and nonalcoholic steatohepatitis are the main risk factors for HCC. The bulk of HCC cases are seen in underdeveloped nations, mainly in East and Southeast Asia and Sub-Saharan Africa, where the hepatitis B virus (HBV) is common [3].
Patients are cured with surgical excision, orthotropic liver transplantation, and local percutaneous tumor ablation. However, these curative options are only available to a limited percentage of patients, because most patients are diagnosed with unresectable cancers at an advanced stage [4]. HCC is commonly misdiagnosed until it has progressed to an advanced stage, with a median survival duration of 3 to 16 months, and most patients are ineligible for the treatment choices listed above [5].
After surgery, chemotherapy, and radiation therapy, immunotherapy is the fourth pillar of cancer treatment. Immune cell therapy, such as chimeric antigen receptor T-cell therapy and checkpoint inhibitors, is unusual in that it uses the host immune system to treat cancer, as opposed to traditional approaches that target cancer cells [6]. Immunological-based methods include cytokines, vaccinations, adoptive cell therapy (based on dendritic cells [DCs]), and immune checkpoint inhibitors [7]. Antigenic chemicals are used in cancer vaccination to activate tumor-specific immune responses that can reduce the tumor load and prevent relapse. Cancer cells, antigen peptides, DCs, and DNA-based vaccines are all used in HCC vaccines, and some effectively prevent tumor recurrence and metastasis [8]. DCs are the most powerful instrument for professional antigen-presenting cells and play a key role in the primary immune response, tolerance, and maintenance of immunological homeostasis. DCs are also an important link between innate and adaptive immune systems [9]. Moreover, DCs can process and present antigens to T cells, which results in the formation of tumor-specific CD8 T cells that detect peptides produced from intracellular proteins and are presented on major histocompatibility complex (MHC) class I complexes, thus resulting in efficient antigen-specific T-cell immunity [10].
Immunotherapy may be a potential treatment for HCC, which is a national health problem in Egypt. The therapeutic effects of DCs on HCC are unknown; to our best knowledge, this is the first study utilizing intradermal injection of autologous DCs in patients with advanced HCC from Egypt. However, the goal of our study was to compare the safety and efficacy of peripheral blood-derived autologous DC injection treatment with other supportive treatments to control the progression and overall prognosis of advanced HCC in Egyptian patients.
Material and methods
Study population
This research was completed at Sohag Center of Cardiac and Digestive System between April 2018 and March 2019, in Sohag, Egypt. The study included 36 patients with advanced HCC who were not eligible for curative treatment (29 males and 7 females). The average age of the participants in the study was 56 years. The mean age of the injection group was 64.76 ±8.22, whereas the mean age of the control group was 66.68 ±7.39 years. This study was approved by the Cardiac and Digestive System Hospital’s review board, and all patients provided written informed consent prior to enrolling in the trial, in accordance with the Helsinki criteria for research ethics.
The inclusion criteria were adults (male or female) aged 18 to 80 years old who were post-hepatitis C virus (HCV) cirrhotic patients with advanced HCC (i.e., patients who were not suitable for conventional lines of treatment) and patients with negative human immunodeficiency virus, human T-lymphotropic virus type 1 and 2, and syphilis. The exclusion criteria of patients were patients with alcoholic liver cirrhosis, autoimmune liver cirrhosis, hepatic transplantation, autoimmune diseases, chronic infectious diseases, or other malignancies, and patients who were pregnant or breastfeeding.
Participants in the study were separated into two groups: Group I included 19 HCC patients (14 males and 5 females) who continued to take supportive medications such as albumin, fresh plasma, and vitamin K, as usual (control group). Group II included 17 HCC patients (15 males and 2 females) who received autologous DC injection therapy.
Clinical, anthropometric, and laboratory measurements
Clinical studies: Full medical history and complete clinical examination for all systems with a stress on the hepatobiliary system, especially ascites, jaundice, previous attacks of hepatic encephalopathy, abdominal pain, and hematemesis.
Laboratory studies: Kidney function tests, liver function tests (total bilirubin, albumin, prothrombin time and concentration, and international normalized ratio [INR]), blood picture test, HCV-Ab, HBsAg, α-fetoprotein (AFP) assay, and immune markers: CD4, CD8, CD16, and CD56.
Imaging: Abdominal ultrasonography was performed to evaluate the liver size, echo pattern, cirrhosis, focal lesions, spleen size, and ascites amount (minimal, moderate, or marked). A triphasic computed tomography scan at the abdomen was performed to confirm the diagnosis of HCC. Clinical assessment, laboratory evaluation (liver function and AFP), and ultrasonographic evaluation were performed 3 and 6 months after injection.
Analytical methods
Liver and renal function tests were performed using a fully automated closed system Vitros 350 analyzer (Ortho-Clinical diagnostics, USA). Viral markers (HBs-Ag and HCV-Ab) were performed using a fully automated closed system mini VIDAS immunoanalyzer (bioMerieux S.A. Clinical Diagnostics, France). AFP was performed using the fully automated closed system Tosoh AIA 360 (TOSOH CORPORATION, Japan). Complete blood count (CBC) was performed using a fully automated closed system Swelab hematology analyzer (Boule Medical AB, Sweden).
Preparation of autologous dendritic cells
Autologous DC injection was prepared following the method of Iwashita et al. [11]. Then, 20 ml of peripheral blood samples was withdrawn with an aseptic technique using preservative-free heparin as an anticoagulant. Mononuclear cells (PBMCs) were isolated by centrifugation of whole blood over a Ficoll-Hypaque density gradient, then mononuclear cells were washed and cultured in T-25 flasks in complete medium [DMEM + 20% autologous serum + 10% antibiotic + 20 ng/ml human interleukin (IL-4) + 50 ng/ml GM-CSF]. Cultures were incubated at 37°C in a humidified CO2 incubator for 7-10 days [12].
Immunophenotyping of cultured DCs
Cultured DCs were stained with fluorescein isothiocyanate (FITC) conjugated mouse mono-clonal antibody (moAb) against CD1a (Dako, Denmark) and with the appropriate isotype-matched control moAb. Cells were incubated with moAb for 30 minutes at 4°C, washed once with phosphate buffered saline (PBS), and re-suspended in a small volume of PBS for analysis with a FACScan flow cytometer (Coulter Epics, Elite). Forward and side scatter gates were established to exclude cell debris and clumps prior to analysis for the expression of the phenotypic marker CD1a [12].
Dendritic cell injection and monitoring of the immunological response
Cells were harvested and activated, and DCs were prepared. Then, treatment was performed twice weekly for three weeks with a total of six vaccinations by intradermal injection of 0.7 IU DCs in the upper third of the arms. Venous blood samples were collected in sterile tubes containing an anticoagulant such as ethylenediaminetetraacetic acid and then stored at room temperature (18°C to 25°C) and not shaken. Before taking the test sample, the samples were homogenized with mild agitation and examined within 24 hours of venipuncture. Flow cytometric assessment of the percentages of CD4, CD8, CD16, and CD56 before injection and one month after injection of DCs was performed using a fully automated closed system flow cytometer (Immunotech S.A.S., a Beckman Coulter Company, France).
Statistical analysis
For statistical data analysis, IBM SPSS version 25 (IBM Corporation, Armonk, USA; August 2017) was utilized. The mean, standard deviation, number, and percentage were used to represent the data. To compare the means of two groups, Student’s t-test was employed, and a one-way analysis of variance test was used to compare the means of more than two groups; the individual p values between each two groups were calculated using the least significant difference post-hoc test. To compare the means, a paired t-test was performed, and the McNemar χ2 test was used to compare the percentages of the same variables over time. To compare two quantitative variables, Pearson’s correlation test was performed. Statistical significance was assigned to values with a p value of less than 0.05.
Results
Table 1 shows the demographic, clinical, and laboratory data of the injected patients (group I) and the control group (group II). In terms of demographic (age and sex) and baseline laboratory data, such as the blood picture, serum albumin, total bilirubin, INR, serum creatinine, AFP, and Child-Pugh score, no statistically significant differences between the two groups were detected. The initial lymphocyte subset enumeration in both groups at baseline showed no statistically significant differences (Table 2).
Table 1
Comparison between the two groups at baseline regarding demographic, clinical, and laboratory data
Table 2
Comparison between the two groups at baseline regarding CDs
All patients received DC injections with no serious adverse events. The most common side effects were injection site rash, mild fever, and headache. There were no changes in vital signs or laboratory results. On monitoring injected patients at 1, 3, and 6 months after DC injection, there were significant differences between the two groups regarding the leukocyte, serum albumin, total bilirubin, and INR levels at both 3 months and 6 months, as shown in Table 3. AFP levels decreased significantly from 232 ng/dl at baseline to 193 ng/dl after 3 months and to 153 ng/dl after 6 months in the vaccinated group, whereas it increased in the control group from 228 ng/dl at baseline to 269 ng/dl at 3 months to 305 ng/dl at 6 months, as shown in Table 3.
Table 3
Comparison between the two groups at 3 and 6 months regarding laboratory, ascites, and Child score
Regarding ascites, only 15.8% of the control cases remained ascites-free after 6 months, as compared to 76.5% in the injected group, while the remaining 85% had different degrees of ascites, with around 42% developing tense ascites at 6 months. The differences between the two groups were significant (p < 0.007) at 3 months and (p < 0.001) at 6 months.
Similar to ascites, most of the cases deteriorated in the control group from class A to B and even nine cases (47.4% of the control group) fell into Child-Pugh class C at the end of the study as compared to 0% in the injected group. The differences between the two groups were significant (p < 0.002) at 3 months and (p < 0.001) at 6 months (Table 3). The median survival time in group I patients receiving DC injection therapy was 7 months compared to 4 months in group II.
Regarding lymphocyte subsets, 1 month after DC injection, there was a highly statistically significant (p < 0.001) difference between the mean values of CD4, CD8, CD16, and CD56 after the injection, as shown in Table 4. CD4+% increased from 27.4% before injection to 38.3% after injection and CD8+% increased from 12.3% before injection to 22.2% after injection. Furthermore, the CD16+% increased from 4.5% before injection to 12.4% after injection and CD56+% decreased from 10.7% before injection to 1.2% after injection (Fig. 1).
Table 4
Comparison between the injection group (before and after) regarding CDs
Fig. 1
Assessment of the percentages of CD4, CD8, CD16, and CD56 before and after injection using a flow cytometer (Beckman Coulter)
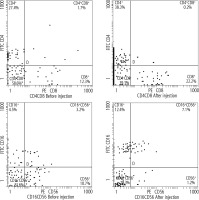
Figure 2 shows that there was a significant positive correlation between AFP at 6 months and CD4% after treatment (r = 0.596, p = 0.012). There was also a considerable positive correlation between AFP at baseline and the CD4/CD8 ratio before treatment (r = 0.401, p = 0.015). On the other hand, there was a significant negative correlation between AFP before and at CD16+% and CD56+% before treatment (r = −0.367, p = 0.028).
Discussion
Chronic viral hepatitis cases, especially patients with HCV and HBV, often fall victim to liver fibrosis and, finally, induced HCC [13]. HCC is one of the most frequently diagnosed cancers, with over 600,000 deaths per year [14]. Because advanced HCC is an aggressive disease, many patients are ineligible for traditional HCC treatments such as resection, transplantation, radiofrequency, or trans-arterial chemo-embolization [15]. After loco-regional therapy, HCC is frequently discovered in an advanced unresectable stage, with recurrence rates as high as 70% [16].
This expresses how alternative treatment options, such as tumor immunotherapy, are viewed. Cancer cells that have undergone genetic changes produce novel antigens, which can be collected and processed by DCs to trigger an anti-tumor response in both T cells and NK cells [17]. DCs, the most powerful and experienced antigen-presenting cells, display MHC classes I and II as well as high quantities of the co-stimulatory molecules CD40, CD80, and CD86. Antigens can be captured, processed, and presented by DCs to activate antigen-specific cytotoxic T lymphocytes. Dendritic cells are also able to activate NK cells, which are essential for induction of the T-lymphocyte response. In addition, activated NK cells directly lyse tumor cells and secrete tumor necrosis factor α (TNF-α) and interferon γ (INF-γ) [18]. Ferlazzo et al. [19] suggested a reciprocal activation cycle between DCs and NK cells whereby NK cells activated by DCs are able to activate new DCs. End-stage patients with B-cell lymphoma, melanoma, renal cell carcinoma, prostate cancer, and other cancers received DC-based immunotherapy in clinical trials [20]. The majority of these studies revealed that DC-based immunotherapy holds promise for cancer elimination [21]. Chen et al. found that DC-based therapy did not increase anti-tumor immunity, improved the survival rate, and extended the survival duration of HCC patients and demonstrated its safety based on 1,276 cases from 19 clinical studies. These are promising results for the future development of DC-based immunotherapy as an adjuvant treatment for HCC [22].
A number of immunotherapy modules against cancer have been introduced to improve the overall survival of patients. Immunotherapy strategies for HCC patients include different mechanisms such as immunosuppressive, immune evasion, effector T-cell dysfunction, immune checkpoint molecules’ expression alterations, and deregulation of cytokines. Immunotherapy is mediated through the use of DC vaccines. So, active immunization with DCs could be highly effective for patients with HCC [23]. Anti-tumor vaccinations, particularly DC immunization, are effective in a number of animal models and clinical trials [24]. To elicit AFP-specific immune responses, HCC patients’ DCs can be transduced with an AFP-expressing adenovirus [25]. DCs with RNA cells from HepG2 tumor cells were also able to produce anti-HCC T lymphocytes [26]. When DCs were loaded with Hsp70-peptide complexes produced from human HCC cells, they matured, thereby stimulating the proliferation of autologous HCC-specific cytotoxic T lymphocytes (CTLs) [27].
The ability of DCs to grab antigens from tumor cells and transport them to draining lymph nodes, where the tumor antigen is presented to T cells, is crucial for the intratumoral injection of DCs in HCC patients [28]. To our knowledge, only a few immunotherapy trials for HCC treatment have been completed, and only a few trials using DCs pulsed with autologous HCC tumors or tumor cell lines have been initiated. DCs are increasingly being used as adjuvants in immunization techniques; however, very little research on DC vaccines for patients with hepatocellular carcinoma has been conducted [29]. Our study’s major objective was to evaluate the safety and efficacy of a peripheral blood-derived autologous DC vaccination to limit tumor growth and determine the overall prognosis for patients with advanced HCC.
The current study was conducted on 36 HCC patients who were randomly assigned to control and DC vaccine groups. The DC vaccine group received an intradermal DC vaccine twice weekly for three weeks with a total of six vaccinations of 0.7 IU. Clinical evaluation of patients after immunotherapy showed statistically significant improvement in ascites (p < 0.001). The Child-Pugh score of the study group showed a statistically significant difference from that of the control group at 6 months’ follow-up.
Laboratory evaluation showed a significant reduction of AFP from 232 ng/dl at baseline to 153 ng/dl after 6 months for the injection group, whereas AFP increased in the control group from 228 ng/dl at baseline to 305 ng/dl at 6 months. These findings were consistent with those of El Ansary et al. [30] who found that three patients had lower serum AFP levels and that 8 out of 10 patients showed AFP-specific immunologic improvements. According to Wang et al. [31] the mean levels of AFP, AFP-L3, alanine aminotransferase (ALT), and CA19.9 were significantly lower after dendritic cells-cytotoxic T lymphocytes (DC-CTL) treatment (p = 0.0437, p = 0.0471, p = 0.0037, and p = 0.0262, respectively) than in pre-treatment patients who received DC-CTL therapy.
Regarding liver function tests, including serum albumin, total bilirubin, and INR improved significantly in the DC injection group, whereas these parameters showed a marked deterioration in the control group compared to baseline values. These findings may be explained by a regressive or stable disease course that prevents further decompensation, which agreed with the findings of El Ansary et al. [30].
Regarding lymphocyte subset enumeration, the present study revealed an increase in both T-cytotoxic and NK cell numbers, which denoted an improvement in anti-tumor cell-mediated immunity. The T-cell subset enumeration revealed a statistically significant increase in T-cytotoxic cells and a reduction in the CD4/CD8 ratio in the study group. This was due to the improved anti-tumor T-cell response after DC vaccination. These findings were parallel with those of Wang et al. [31], who discovered that the percentages of CD3+CD4+ T cells in the peripheral blood decreased significantly (p > 0.0001), whereas the number of CD3+CD8+ T lymphocytes tended to increase (p > 0.0001). Also, Butterfield et al. [32] verified this notion by detecting AFP-specific CD8+ cell responses in HCC patients receiving AFP-peptide immunization. According to Chen et al., the CD4+T/CD8+T ratio reflects the level of anti-tumor immunity to some extent. As a result, alterations in anti-tumor immune activity in HCC patients after DC-based treatment can be reflected by CD4+T/CD8+T [22].
Dendritic cell vaccinations produced antigen-specific cytotoxic T-lymphocyte and T helper 1 responses in healthy volunteers and patients with a variety of advanced malignancies, according to van der Burg [33]. CTLs co-cultured with the HepG2 cell line produced more IFN-γ than CTLs co-cultured with the SMMC7721 and K562 cell lines, according to Zhang et al. [34].
The other arm of the cell-mediated anti-tumor response is NK cells. In this study, the number of NK cells showed a significant increase in the study group over the control group after DC vaccination [35]. This was in accordance with the hypothesis of Hu et al. [36], who proposed that a major arm of the anti-tumor response elicited by DCs may occur through the activation of NK cells.
To the best of our knowledge, this is the first clinical study to evaluate the role of the DC vaccine in NK activation. The limitations of our work were that it is a single-center experience using a small sample of patients, which requires validation in other centers with patient follow-up.
In conclusion, we developed a protocol for harvesting high-quality sufficient DCs and studied the effects of DC injection therapy on patients with HCC. Our results revealed a significant reduction in HCC markers as well as several specific clinical indexes, including AFP, ALT, creatinine, bilirubin, INR, albumin, and CBC parameters. Therefore, DC injectable treatment may help patients with invasive malignancies maintain or improve their quality of life. These findings indicate the potential involvement of DC injection therapy in the treatment of HCC patients.