Purpose
Intra-cavitary brachytherapy (ICB) is an essential component of definitive radiotherapy for cervical cancer treatment. Although extra beam radiotherapy (EBRT) has developed remarkably in recent years, advanced EBRT, such as intensity-modulated radiotherapy or stereotactic body radiotherapy, is not recommended as an alternative to brachytherapy [1, 2]. Three-dimensional image-guided adaptive brachytherapy (3D-IGABT) has been standardized by working groups in the United States and Europe in the last decades [3-7]. The 3D-IGABT enables accurate dose assessment, and shows higher local control and lower toxicity than two-dimensional image-based brachytherapy [8-11]. However, gastro-intestinal toxicity in cervical cancer radiotherapy compromises the quality of life if it occurs. A recent large-scale prospective cohort study of 3D-IGABT reported that morbidity of grade 3 or higher gastro-intestinal toxicity account for 8.5% of the cases [12], and correlate with the irradiated dose to the rectum or bowel [13].
Various methods, such as rectal retraction (RR), vaginal gauze packing (VP), inflatable catheter, or posterior vaginal speculum blade, have been used to displace the rectum away from the applicator and reduce the rectal dose in brachytherapy [14]. Of these, RR and VP were the most prevalent. Several studies have shown that RR combined with tandem and ring applicator (TR) achieves a lower rectal dose than VP alone [15-17]. However, few studies have compared RR and VP in terms of tandem and ovoid applicator (TO) only. TO is still mainly used instead of TR, especially in Asian countries [18-21]. The irradiated volume is larger and the dose to the rectum is higher with a TO than with a TR [19, 22-24]. Therefore, the dose reduction effect of each RR method can differ with the use of a TO. We conducted the present study to assess the impact of RR and VP in combination with a TO on rectal dose. We also assessed the difference in the dose to other organs at risk (OARs), such as the sigmoid colon, small bowel, and bladder.
Material and methods
Ethics statements
The institutional research ethics board of our university approved the retrospective analysis of patient dosimetric and clinical data (approval number, 20200176).
Study design and patient population
Patients with cervical cancer treated with definitive (chemo)radiotherapy, including ICB with tandem and ovoid applicators, between April 2019 and March 2022 at our hospital, were retrospectively reviewed. We analyzed patients who underwent ICB with both RR and VP in at least one fraction. Patients who were not treated with a TO but with other methods, such as tandem and cylinder applicator, those who could not be adequately inserted with RR, or were treated with an interstitial needle, were excluded from analysis.
Radiotherapy
All patients received 50-50.4 Gy in 25-28 fractions (1.8-2.0 Gy/fraction) of whole pelvis irradiation (WPRT) with or without central shielding (CS), and 12-24 Gy in 2-4 fractions (6 Gy/fraction) of high-dose-rate ICB once a week according to the Japanese Society of Gynecologic Oncology guidelines [25]. Patients who were inserted with CS after 30 or 30.6 Gy of WPRT received 4 fractions of ICB. Patients who were inserted with CS after 40 or 41.4 Gy of WPRT received 3 fractions. Patients who were not inserted with CS received 2-3 fractions. ICB was proceeded after completion of WPRT (and concurrently with CS for inserted patients).
Brachytherapy procedure
Brachytherapy was performed with the patient under conscious sedation with intravenous midazolam if there were no contraindications. The patient’s bladder volume was verified by trans-abdominal ultrasonography (TAUS) before procedure. In patients with insufficient volume, the bladder was catheterized and inflated with saline. A tandem applicator was inserted under the guidance of TAUS. For the first one or two fractions, VP was applied. A metallic applicator with half ovoids was routinely used in the VP group (Figure 1A). RR was applied in later fractions for patients who were assumed to have RR adequately inserted. A resinous applicator with full ovoids was used in the RR group (Figure 1B, C). Computed tomography (CT) images and dose distributions of representative cases from both arms are shown in Figure 2A-D.
Fig. 1
Tandem and ovoid applicators used in this study. A) A metallic applicator with vaginal gauze packing. B, C) A resinous applicator with a rectal retractor
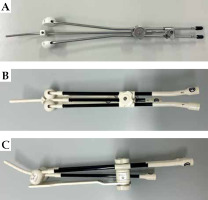
Fig. 2
Computed tomography images and dose distribution of representative cases from the vaginal gauze packing (VP) and rectal retractor (RR) groups. A) Axial image from VP group. B) Sagittal image from VP group. C) Axial image from RR group. D) Sagittal image from RR group
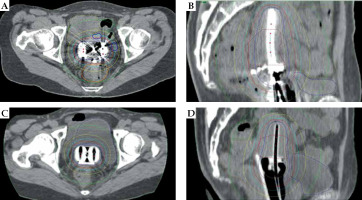
CT images were obtained after applicator insertion at each fraction. Treatment plans were generated using Oncentra Brachy (Elekta, Stockholm, Sweden) based on CT image findings. Magnetic resonance images were taken at diagnosis and immediately before the first ICB for target delineation. High-risk clinical target volume (HR-CTV) was determined based on recommendations for CT-based IGBT from Japan [26]. The rectum, sigmoid, small bowel, and bladder were delineated as OARs. Dose distribution was manually optimized to meet dose constraints for HR-CTV and OARs. The total doses of EBRT and each ICB fractions were calculated as cumulative linear quadratic equivalent dose (EQD2) using α/β = 10 Gy for HR-CTV, and α/β = 3 Gy for OARs. The planning aim was > 70 Gy for HR-CTV D90 (dose to 90% of HR-CTV), < 75 Gy for rectum and sigmoid D2cm3 (minimum dose to the most exposed 2.0 cm3 of OARs), and < 90 Gy for bladder D2cm3 based on guidelines from working groups in the United States and Europe and a protocol of a prospective multi-institutional study from Japan [4, 6, 27].
Data collection and statistical analysis
Dose-volume parameters for HR-CTV (D90), rectum (D0.1cm3, D2cm3), sigmoid colon (D0.1cm3, D2cm3), small bowel (D2cm3), and bladder (D0.1cm3 and D2cm3) were collected. First, VP and RR plans were compared, which are actually used in clinical practice. Second, to verify the unmitigated impacts of rectal retraction methods, VP and RR plans normalized by D90 of HR-CTV of corresponding VP plans were compared. Each dose-volume parameter was compared between the VP and RR groups using Wilcoxon’s rank-sum test. Moreover, the correlation between the impact of rectal D2cm3 and patient characteristics, such as body mass index (BMI) and HR-CTV at first brachytherapy, was evaluated.
All statistical analyses were performed using SPSS statistics, version 28.0.1.0 (IBM Corp., Armonk, New York, USA). A p-value of ≤ 0.05 was considered significant in all statistical analyses.
Results
In total, 136 patients received definitive radiotherapy, including ICB, between April 2019 and March 2022. Among them, 63 patients who underwent ICB with VP only, 13 patients who underwent intra-cavitary/interstitial brachytherapy, 9 patients who were treated with tandem and cylinder applicators, and 1 patient whose treatment was discontinued due to a serious infection, were excluded. Finally, 50 patients were included in the present study, and 100 procedures were analyzed. Patient characteristics are shown in Table 1.
Table 1
Patient characteristics (n = 50)
Dose-volume parameters of the VP and non-normalized RR groups are listed in Table 2. The D0.1cm3 (5.798 Gy vs. 6.116 Gy, p = 0.048) and D2cm3 (4.234 Gy vs. 4.627 Gy, p = 0.008) of the rectum were significantly lower in the VP group than in RR group. The D0.1cm3 (7.639 Gy vs. 8.407 Gy, p = 0.014) and D2cm3 (5.959 Gy vs. 6.690 Gy, p < 0.001) of the bladder were significantly lower in the VP group than in RR group. There was no significant difference in dose-volume parameters of the sigmoid colon and small bowel. The D90 of HR-CTV tended to be lower in the VP group than in RR group (7.479 Gy vs. 7.652 Gy, p = 0.172). HR-CTV values were 28.79 cm3 in the VP group and 27.12 cm3 in RR group. The HR-CTV tended to be larger in the VP group than in RR group, but there was no significant difference (average: 28.79 cm3 in the VP group, and 27.12 cm3 in RR group, p = 0.212).
Table 2
Comparison of DVH parameters between VP and non-normalized RR groups
[i] VP – vaginal gauze packing, RR – rectal retractor, SD – standard deviation, HR-CTV – high-risk clinical target volume, D0.1cm3 – minimum dose to most exposed 0.1 cm3 of organs at risk, D2cm3 – minimum dose to most exposed 2 cm3 of organs at risk, D90 – minimum dose to 90% of high-risk clinical target volume
The dose-volume parameters of the VP and RR groups normalized by the D90 of HR-CTV are presented in Table 3. The D2cm3 of the rectum was significantly lower in the VP group than in RR group (4.234 Gy vs. 4.543 Gy, p = 0.049). The D2cm3 (5.959 Gy vs. 6.546 Gy, p = 0.006) of the bladder was significantly lower in the VP group than in RR group. There was no significant difference in dose-volume parameters of the sigmoid, bowel, and HR-CTV.
Table 3
Comparison of DVH parameters between VP and normalized RR groups
[i] VP – vaginal gauze packing, RR – rectal retractor, SD – standard deviation, HR-CTV – high-risk clinical target volume, D0.1cm3 – minimum dose to most exposed 0.1 cm3 of organs at risk, D2cm3 – minimum dose to most exposed 2 cm3 of organs at risk, D90 – minimum dose to 90% of high-risk clinical target volume
In patients with an HR-CTV < 23.21 cm3 (i.e., the median in this cohort) at first brachytherapy, the D2cm3 of the rectum was significantly lower in the VP group than in non-normalized RR group (3.727 Gy vs. 4.290 Gy, p = 0.011) and normalized RR group (3.727 Gy vs. 4.354 Gy, p = 0.014). In patients with a BMI < 22.04 kg/m2 (i.e., the median in this cohort), the D2cm3 of the rectum was significantly lower in the VP group than in non-normalized RR group (4.317 Gy vs. 4.850 Gy, p = 0.009) and normalized RR group (4.317 Gy vs. 4.856 Gy, p = 0.016). In patients with a larger HR-CTV volume or BMI, there was no significant difference (Table 4).
Table 4
Rectal dose according to HR-CTV and BMI in VP and normalized RR groups
Discussion
In the current study, we demonstrated that VP achieved a lower rectal dose than RR in tandem and ovoid ICB, especially in patients with smaller CTV or lower BMI. This study indicates that VP can reduce rectal toxicity correlated with the irradiated dose to the rectum, at least in a certain population.
One of the strengths of the study was the comparison of RR with VP in the same patients. The dose to OARs or targets is influenced by several factors, except for VP or RR, such as the clinical target volume (CTV), FIGO stage, and BMI [19, 28]. A comparison of the same patients can reduce this bias. The second strength of this study was the use of 3D-IGABT, which achieves a lower dose to OARs without impairing the dose to CTV than two-dimensional image-based brachytherapy [10]. The effect of RR or VP on the rectal dose reduction may be compromised by an optimization. This study’s findings show the advantages of VP in a 3D-IGABT setting. The third strength of this study is the normalization of the dose to HR-CTV. A previous study showed that dose to point A or D90 are factors affecting the rectal dose [15]. In the present study, VP was used at an earlier fraction than RR; hence, the HR-CTV was larger and the dose to HR-CTV was lower than those of the RR group. To minimize such bias, the dose-volume parameter of the RR group was normalized, such that D90 was equivalent to that of the VP group. We demonstrated the superiority of VP with or without normalization.
Patil et al. compared VP and RR use in the same patients, and showed a lower dose to the rectum and bladder in the VP group [29]. Even though the details of this study are unknown because we could refer only to the abstract, the results are similar to these in the present study. Dasgupta et al. compared VP and RR use in tandem and ovoid ICB, and found no differences [30]. This may be because this study did not contain a sufficiently large cohort to show statistical significance.
Several previous studies have demonstrated a better rectal dose reduction effect of RR than VP [15-17]. The present study appears to contradict these previous studies’ findings. One possible reason for this is the difference in dose distribution between TO and TR. The irradiated volume and dose to the rectum with TO are larger than that with TR [19, 22-24]. Differences in dose distribution by applicators may lead to differences in rectal dose reduction effects. Another reason is that more gauze can be packed in the ventral part of the rectum with TO than with TR. With TO, there is a larger space to pack the gauze between ovoids than with TR.
The dose administered to the bladder was significantly lower in the VP group than in RR group. This is thought to be due to VP between the tandem and bladder in the VP group; in contrast, there was nothing to displace the bladder away from the tandem in the RR group. This result corresponds to that of a previous report [29]. The dose to the sigmoid colon tended to be lower in the VP group than in RR group, but the difference was not significant. The superiority of VP or RR in reducing dose to the sigmoid colon is not clear in previous studies on TR [15-17]. A earlier study comparing VP and balloon packing showed no significant results in the dose to the sigmoid [31]. Rectal dose reduction methods may not significantly affect the dose to the sigmoid colon.
In the sub-group analysis, we showed a greater advantage with VP in patients with a smaller CTV or lower BMI. This tendency corresponds with findings from a study that reported the superiority of VP in India [29], where the population’s BMI is low [32]. Patients with a smaller CTV and lower BMI often have a smaller vaginal vault and little perineal fat. These factors improve visibility of the procedure and facilitate adequate packing of gauze. We believe this is the reason for the greater dose-reductive effect of VP in this population.
The present study has some limitations. First, it was a retrospective analysis of a small number of patients. Second, the presented comparison was only a dose-volume histogram of the treatment planning system. Herein, a metallic applicator and gauze with a radiopaque marker were applied in the VP group, whereas a resinous applicator with no gauze was used in the RR group. This difference induced more uncertainty in delineation due to metallic artifacts on CT in the VP group. Uncertainty of delineation impacts the dose to the target and OARs [33-35]. Third, VP has poorer reproducibility than RR. The number and position of gauze are highly dependent on radiation oncologist’s experience. A previous study indicated that inappropriate packing causes lower disease control and more toxicity [36]. Therefore, this study’s results may not be applicable to all facilities. A prospective study to assess clinical outcomes is needed to verify the benefit of VP over RR.
Conclusions
Here, we demonstrated the superiority of VP in reducing the dose to the rectum and bladder compared with RR. The impact of VP tended to be greater in patients with a smaller CTV and lower BMI. Although the superior method may differ according to patient characteristics, disease status, or the experience of radiation oncologist, VP is still a promising method to reduce the dose to the rectum and bladder.